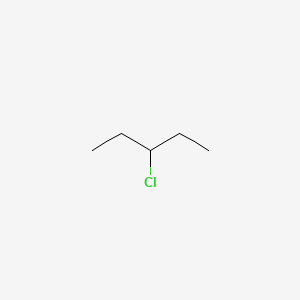
3-Chloropentane
Overview
Description
3-Chloropentane (C₅H₁₁Cl, CAS 616-20-6) is a secondary chloroalkane with a chlorine atom attached to the third carbon of a pentane chain. Its IUPAC name is this compound, and its molecular weight is 106.59 g/mol. Key physical properties include a boiling point of 97.55°C, density of 0.8854 g/cm³, and refractive index of 1.4059 . The compound is synthesized via free radical chlorination of pentane, which produces a mixture of 1-, 2-, and this compound isomers . Unlike its isomers, this compound lacks a chiral center due to the symmetrical substitution pattern at the C3 position (attached to two CH₂ groups), rendering it achiral .
Preparation Methods
Synthetic Routes and Reaction Conditions: 3-Chloropentane can be synthesized through the free-radical chlorination of pentane. This process involves the substitution of a hydrogen atom in pentane with a chlorine atom, typically using chlorine gas and ultraviolet light to initiate the reaction .
Industrial Production Methods: In an industrial setting, this compound can be produced by the reaction of 3-pentanol with hydrochloric acid in the presence of anhydrous zinc chloride. Another method involves treating 3-pentanol with phosphorous trichloride in excess dimethylformamide (DMF) .
Chemical Reactions Analysis
Nucleophilic Substitution Reactions
3-Chloropentane undergoes both SN1 and SN2 mechanisms, depending on reaction conditions:
SN2 Mechanism
-
Conditions : Polar aprotic solvents (e.g., acetone), strong nucleophiles (e.g., OH⁻, CN⁻).
-
Example : Reaction with sodium hydroxide yields 3-pentanol via a bimolecular transition state .
SN1 Mechanism
-
Conditions : Polar protic solvents (e.g., water/ethanol), weak nucleophiles.
-
Example : Hydrolysis in aqueous ethanol forms 3-pentanol through a carbocation intermediate .
Mechanism | Solvent | Nucleophile | Major Product | By-Products |
---|---|---|---|---|
SN2 | Acetone | OH⁻ | 3-Pentanol | None |
SN1 | H₂O/EtOH | H₂O | 3-Pentanol | Minor alkenes |
Elimination Reactions
Under basic conditions, this compound undergoes E1 or E2 elimination to form alkenes:
E2 Elimination
-
Conditions : Strong bases (e.g., KOtBu), heat.
-
Product : Predominantly pent-2-ene (Zaitsev product) .
E1 Elimination
-
Conditions : Weak bases (e.g., H₂O), protic solvents.
-
Product : Mixture of 1-pentene and 2-pentene , with the latter as the major product .
Mechanism | Base | Temperature | Major Product (%) | Minor Product (%) |
---|---|---|---|---|
E2 | KOtBu | 80°C | 2-Pentene (85%) | 1-Pentene (15%) |
E1 | H₂O | 25°C | 2-Pentene (70%) | 1-Pentene (30%) |
Radical Substitution
Free-radical chlorination of pentane produces this compound as a minor product due to the stability of secondary radicals .
Reactant | Conditions | Major Products | Yield (this compound) |
---|---|---|---|
Pentane | Cl₂, UV light, 25°C | 1-Chloropentane (45%), 2-Chloropentane (35%) | 20% |
Competition Between Substitution and Elimination
Reactions often yield mixtures of substitution and elimination products. For example:
-
This compound + NaOH (aq) : 70% 3-pentanol (SN2), 30% pent-2-ene (E2) .
-
This compound + H₂O/EtOH : 55% 3-pentanol (SN1), 45% alkenes (E1) .
Key Mechanistic Insights
-
Steric Effects : The central chlorine atom in this compound reduces steric hindrance, favoring SN2 over branched chloroalkanes.
-
Carbocation Stability : In SN1/E1 pathways, the secondary carbocation intermediate facilitates faster reactions compared to primary analogs.
Scientific Research Applications
Organic Synthesis
Intermediate in Chemical Reactions
3-Chloropentane serves as a crucial intermediate in the synthesis of more complex organic molecules. It can undergo various chemical reactions, including:
- Nucleophilic Substitution Reactions : Here, the chlorine atom is replaced by a nucleophile, such as hydroxide ions, leading to the formation of alcohols or ethers.
- Elimination Reactions : Under strong basic conditions, this compound can lose hydrogen chloride (HCl) to form alkenes, such as pent-2-ene.
Reaction Pathways
Reaction Type | Example Product | Conditions |
---|---|---|
Nucleophilic Substitution | Alcohols/Ethers | NaOH/KOH in aqueous/alcoholic medium |
Elimination | Pent-2-ene | Strong bases like KOtBu |
Pharmaceutical Research
Drug Development
this compound has been investigated for its potential in drug development, particularly in creating compounds with biological activity. Notable pharmacological effects include:
- Anticholinesterase Activity : Compounds derived from this compound have shown significant inhibition of acetylcholinesterase (AChE), which is relevant for treating neurodegenerative diseases like Alzheimer's.
Case Study: Anticholinesterase Activity Assessment
A study evaluated the anticholinesterase activity of derivatives synthesized from this compound. The following table summarizes the findings:
Compound | Concentration (mM) | AChE Inhibition (%) |
---|---|---|
Compound A | 0.1 | 45 |
Compound B | 1.0 | 75 |
Control | - | 10 |
This data indicates that certain derivatives exhibit promising AChE inhibition compared to controls.
Material Science
Production of Specialty Chemicals
In material science, this compound is employed in synthesizing specialty chemicals used in various applications. Its unique reactivity allows for the creation of polymers and other materials that have specific desired properties.
Research indicates that derivatives of this compound possess notable biological activities, including:
- Antimicrobial Properties : Certain thiazole derivatives synthesized from this compound exhibit antimicrobial activity against pathogens like Escherichia coli and Salmonella species.
Research Findings on Antimicrobial Activity
The following table presents the antimicrobial efficacy of thiazole derivatives:
Bacterial Strain | Inhibition Zone (mm) | Control (Gentamicin) (mm) |
---|---|---|
Escherichia coli | 18 | 22 |
Salmonella sp. | 15 | 20 |
Candida albicans | 12 | 16 |
These results suggest that certain derivatives may offer comparable antimicrobial activity to established treatments.
Mechanism of Action
The mechanism of action of 3-Chloropentane primarily involves radical substitution and nucleophilic substitution reactions. In radical substitution, the chlorine atom is replaced by a radical species, while in nucleophilic substitution, the chlorine atom is replaced by a nucleophile. These reactions typically proceed through the formation of a carbocation intermediate .
Comparison with Similar Compounds
Structural and Physical Properties
The table below compares 3-chloropentane with its structural isomers:
Property | 1-Chloropentane | 2-Chloropentane | This compound |
---|---|---|---|
Boiling Point (°C) | 108.2 | 97.6 | 97.55 |
Density (g/cm³) | 0.883 | 0.880 | 0.8854 |
Chirality | No | No | No |
Reactivity | Primary chloride | Secondary chloride | Secondary chloride |
Data indicate minimal differences in boiling points and densities among isomers, but reactivity diverges significantly due to the position of the chlorine atom .
Reactivity in Friedel-Crafts Alkylation
This compound participates in AlCl₃-catalyzed alkylation of arenes but exhibits distinct behavior compared to 2-chloropentane:
- Product Distribution : At −20°C, alkylation of benzene with this compound yields an initial 65:35 ratio of 3-:2-phenylpentane, shifting to 68:32 over time. In contrast, 2-chloropentane produces a stable 74:26 ratio of 2-:3-phenylpentane under the same conditions .
- Reaction Rates : The alkylation rate of 2-chloropentane is 2–3 times faster than that of this compound due to the greater stability of the secondary carbocation intermediate formed from 2-chloropentane .
- Rearrangement: Carbocation intermediates from this compound undergo partial isomerization to 2-pentyl carbocations, leading to mixed products. For example, heating 3-pentanol with HCl generates ~67% 2-chloropentane and 33% this compound .
Stereochemical and Spectroscopic Differences
- Chirality : Unlike 3-chloro-1-pentene (chiral due to asymmetric substitution at C3), this compound is achiral .
- IR Spectroscopy : The C-Cl stretching frequency in this compound (667 cm⁻¹ in certain conformations) differs from 1- and 2-chloropentane due to conformational effects .
Key Research Findings
Table 1: Alkylation Product Distributions with Different Chloropentanes
Substrate | Temperature | Product Ratio (2-:3-phenylpentane) | Reference |
---|---|---|---|
2-Chloropentane | 25°C | 74:26 | |
This compound | 25°C | 74:26 (after equilibration) | |
This compound | −20°C | 65:35 → 68:32 (over 2.5 h) |
Table 2: Relative Alkylation Rates (AlCl₃ Catalyst)
Chloroalkane | Relative Rate (vs. This compound) | Reference |
---|---|---|
2-Chlorohexane | 2–3× faster | |
3-Chlorohexane | 1× (baseline) |
Biological Activity
3-Chloropentane, a chlorinated alkane, has garnered attention in the field of medicinal chemistry due to its diverse biological activities. This compound exhibits significant interactions with various biological targets, particularly in the context of enzyme inhibition and potential therapeutic applications. This article explores the biological activity of this compound, focusing on its mechanism of action, pharmacological effects, and relevant studies.
Chemical Structure and Properties
This compound has the molecular formula and a molecular weight of approximately 134.56 g/mol. Its structure includes a chlorine atom attached to the third carbon of a pentane chain, which contributes to its unique chemical reactivity and biological interactions.
Target Enzymes
The primary biological target of this compound derivatives is cholinesterase enzymes, specifically acetylcholinesterase (AChE) and butyrylcholinesterase (BChE). Inhibition of these enzymes leads to increased levels of acetylcholine in the nervous system, affecting cholinergic signaling pathways.
Mode of Action
The compound interacts with cholinesterase enzymes through reversible or irreversible binding, depending on the specific derivative and experimental conditions. This interaction can lead to enhanced neurotransmission, making it a candidate for further investigation in neuropharmacology .
Pharmacological Effects
This compound has demonstrated various pharmacological effects:
- Anticholinesterase Activity : Several studies have confirmed that this compound derivatives exhibit significant anticholinesterase activity. For instance, compounds synthesized from this compound have shown promising results in inhibiting AChE activity in vitro, which is crucial for developing treatments for conditions like Alzheimer's disease .
- Antimicrobial Properties : Research indicates that thiazole derivatives synthesized from this compound exhibit antimicrobial activity against various pathogens, including Escherichia coli and Salmonella species. These findings suggest potential applications in treating bacterial infections .
- Cardioprotective Effects : Certain derivatives have been tested for their cardioprotective properties using isolated rat thoracic aorta rings. These studies revealed that some compounds derived from this compound could delay constrictor responses, indicating potential benefits for cardiovascular health .
Case Study: Anticholinesterase Activity Assessment
A study conducted to evaluate the anticholinesterase activity of a series of compounds derived from this compound involved measuring the inhibition of AChE using spectrophotometric methods. The results indicated that specific concentrations of these compounds significantly inhibited enzyme activity compared to control groups.
Compound | Concentration (mM) | AChE Inhibition (%) |
---|---|---|
Compound A | 0.1 | 45 |
Compound B | 1.0 | 75 |
Control | - | 10 |
This table illustrates the effectiveness of different derivatives in inhibiting AChE activity.
Research Findings on Antimicrobial Activity
In vitro studies assessed the antimicrobial efficacy of thiazole derivatives synthesized from this compound against various bacterial strains. The results demonstrated significant inhibition zones compared to standard antibiotics.
Bacterial Strain | Inhibition Zone (mm) | Control (Gentamicin) (mm) |
---|---|---|
Escherichia coli | 18 | 22 |
Salmonella sp. | 15 | 20 |
Candida albicans | 12 | 16 |
These findings indicate that certain derivatives possess comparable antimicrobial activity to established treatments.
Q & A
Basic Research Questions
Q. What are the established synthetic routes for 3-Chloropentane, and how do their efficiencies compare under different reaction conditions?
Methodological Answer: this compound is typically synthesized via free radical chlorination of pentane or nucleophilic substitution reactions. For example, chlorination of pentane under UV light yields a mixture of isomers, with this compound as a minor product due to steric factors. Alternatively, SN2 reactions using 3-pentanol and HCl in the presence of ZnCl₂ can improve selectivity. Efficiency varies with temperature, catalyst (e.g., AlCl₃), and reactant ratios. Gas chromatography (GC) is recommended for purity analysis .
Q. What are the key physicochemical properties of this compound, and how do they influence its reactivity in organic reactions?
Methodological Answer: Critical properties include its molecular weight (106.59 g/mol), boiling point (32.9 mmHg at 25°C), and dipole moment (1.5 D). The chlorine atom at the tertiary carbon creates steric hindrance, reducing nucleophilic substitution (SN2) reactivity compared to primary chloroalkanes. However, this position favors elimination (E2) or radical reactions. Computational tools like density functional theory (DFT) can predict reactivity trends .
Q. What safety protocols are essential when handling this compound in laboratory settings?
Methodological Answer: this compound is harmful if ingested or inhaled. Safety protocols include using fume hoods, nitrile gloves, and eye protection. In case of exposure, immediate rinsing with water and medical consultation are advised. Toxicity data (e.g., LD₅₀) should be reviewed from safety data sheets (SDS), and waste must be disposed via halogenated solvent protocols .
Advanced Research Questions
Q. How do reaction temperature and catalyst addition influence isomerization rates of this compound during Friedel-Crafts alkylation?
Methodological Answer: At −20°C, this compound undergoes limited isomerization, favoring direct alkylation of benzene to yield 3-phenylpentane. However, at 25°C, isomerization to 2-Chloropentane occurs, altering product ratios (e.g., 74:26 2-:3-phenylpentane). Adding fresh AlCl₃ shifts equilibrium by regenerating active catalyst sites. Kinetic studies using GC-MS and isotopic labeling can track isomerization pathways .
Q. What mechanistic insights explain the divergent alkylation outcomes between this compound and its structural isomers in aromatic systems?
Methodological Answer: The tertiary chlorine in this compound leads to carbocation formation via SN1 mechanisms, which rearranges less readily than secondary carbocations from 2-Chloropentane. In p-xylene alkylation, steric effects from the methyl group favor 3-p-xylylpentane (48:52 ratio initially), but prolonged reaction times equilibrate products. Transition state analysis via computational modeling (e.g., Gaussian) is critical for elucidating steric and electronic effects .
Q. How can discrepancies in reported isomer ratios from alkylation studies using this compound be resolved?
Methodological Answer: Contradictions arise from variations in catalyst aging, reaction time, and analytical methods. For example, GC retention times may overlap for isomers, requiring tandem MS for resolution. Reproducibility requires strict control of AlCl₃ moisture content and reaction quenching protocols. Meta-analyses comparing data from multiple studies (e.g., Table II in ) can identify systematic errors .
Q. What statistical methods are recommended for analyzing kinetic data from this compound isomerization reactions?
Methodological Answer: Pseudo-first-order kinetics models are applied to isomerization rates. Linear regression of ln([isomer] vs. time) provides rate constants. For competitive alkylation/isomerization systems, the A₂/I₂ ratio (alkylation vs. isomerization rates) is calculated using time-resolved GC data. Bayesian statistical approaches improve confidence intervals in low-yield reactions .
Q. Data Contradiction & Validation
Q. How do solvent polarity and Lewis acid strength affect the regioselectivity of this compound in cross-coupling reactions?
Methodological Answer: Polar aprotic solvents (e.g., DMF) stabilize carbocations, enhancing SN1 pathways, while nonpolar solvents favor radical intermediates. Stronger Lewis acids (e.g., FeCl₃ vs. AlCl₃) increase electrophilicity but may promote side reactions. Validating regioselectivity requires comparative studies with controlled solvent and catalyst variables, analyzed via NMR and IR spectroscopy .
Q. Why do some studies report negligible product isomerization at low temperatures, while others observe dynamic equilibria?
Methodological Answer: At −20°C, product isomerization is kinetically inhibited, but residual catalyst activity may allow slow equilibration. For example, 3-p-xylylpentane remains stable unless fresh AlCl₃ is added. In-situ monitoring via cryogenic NMR or stopped-flow techniques can capture transient intermediates and validate stability claims .
Q. Experimental Design Recommendations
- Control Variables: Document catalyst batch, solvent purity, and quenching methods to ensure reproducibility.
- Analytical Validation: Use hyphenated techniques (GC-MS, LC-NMR) to resolve co-eluting isomers.
- Computational Support: Pair experimental data with molecular dynamics simulations to rationalize steric/electronic effects.
Properties
IUPAC Name |
3-chloropentane | |
---|---|---|
Source | PubChem | |
URL | https://pubchem.ncbi.nlm.nih.gov | |
Description | Data deposited in or computed by PubChem | |
InChI |
InChI=1S/C5H11Cl/c1-3-5(6)4-2/h5H,3-4H2,1-2H3 | |
Source | PubChem | |
URL | https://pubchem.ncbi.nlm.nih.gov | |
Description | Data deposited in or computed by PubChem | |
InChI Key |
CXQSCYIVCSCSES-UHFFFAOYSA-N | |
Source | PubChem | |
URL | https://pubchem.ncbi.nlm.nih.gov | |
Description | Data deposited in or computed by PubChem | |
Canonical SMILES |
CCC(CC)Cl | |
Source | PubChem | |
URL | https://pubchem.ncbi.nlm.nih.gov | |
Description | Data deposited in or computed by PubChem | |
Molecular Formula |
C5H11Cl | |
Source | PubChem | |
URL | https://pubchem.ncbi.nlm.nih.gov | |
Description | Data deposited in or computed by PubChem | |
DSSTOX Substance ID |
DTXSID3060661 | |
Record name | Pentane, 3-chloro- | |
Source | EPA DSSTox | |
URL | https://comptox.epa.gov/dashboard/DTXSID3060661 | |
Description | DSSTox provides a high quality public chemistry resource for supporting improved predictive toxicology. | |
Molecular Weight |
106.59 g/mol | |
Source | PubChem | |
URL | https://pubchem.ncbi.nlm.nih.gov | |
Description | Data deposited in or computed by PubChem | |
CAS No. |
616-20-6 | |
Record name | 3-Chloropentane | |
Source | CAS Common Chemistry | |
URL | https://commonchemistry.cas.org/detail?cas_rn=616-20-6 | |
Description | CAS Common Chemistry is an open community resource for accessing chemical information. Nearly 500,000 chemical substances from CAS REGISTRY cover areas of community interest, including common and frequently regulated chemicals, and those relevant to high school and undergraduate chemistry classes. This chemical information, curated by our expert scientists, is provided in alignment with our mission as a division of the American Chemical Society. | |
Explanation | The data from CAS Common Chemistry is provided under a CC-BY-NC 4.0 license, unless otherwise stated. | |
Record name | Pentane, 3-chloro- | |
Source | ChemIDplus | |
URL | https://pubchem.ncbi.nlm.nih.gov/substance/?source=chemidplus&sourceid=0000616206 | |
Description | ChemIDplus is a free, web search system that provides access to the structure and nomenclature authority files used for the identification of chemical substances cited in National Library of Medicine (NLM) databases, including the TOXNET system. | |
Record name | Pentane, 3-chloro- | |
Source | EPA Chemicals under the TSCA | |
URL | https://www.epa.gov/chemicals-under-tsca | |
Description | EPA Chemicals under the Toxic Substances Control Act (TSCA) collection contains information on chemicals and their regulations under TSCA, including non-confidential content from the TSCA Chemical Substance Inventory and Chemical Data Reporting. | |
Record name | Pentane, 3-chloro- | |
Source | EPA DSSTox | |
URL | https://comptox.epa.gov/dashboard/DTXSID3060661 | |
Description | DSSTox provides a high quality public chemistry resource for supporting improved predictive toxicology. | |
Record name | 3-chloropentane | |
Source | European Chemicals Agency (ECHA) | |
URL | https://echa.europa.eu/substance-information/-/substanceinfo/100.009.517 | |
Description | The European Chemicals Agency (ECHA) is an agency of the European Union which is the driving force among regulatory authorities in implementing the EU's groundbreaking chemicals legislation for the benefit of human health and the environment as well as for innovation and competitiveness. | |
Explanation | Use of the information, documents and data from the ECHA website is subject to the terms and conditions of this Legal Notice, and subject to other binding limitations provided for under applicable law, the information, documents and data made available on the ECHA website may be reproduced, distributed and/or used, totally or in part, for non-commercial purposes provided that ECHA is acknowledged as the source: "Source: European Chemicals Agency, http://echa.europa.eu/". Such acknowledgement must be included in each copy of the material. ECHA permits and encourages organisations and individuals to create links to the ECHA website under the following cumulative conditions: Links can only be made to webpages that provide a link to the Legal Notice page. | |
Retrosynthesis Analysis
AI-Powered Synthesis Planning: Our tool employs the Template_relevance Pistachio, Template_relevance Bkms_metabolic, Template_relevance Pistachio_ringbreaker, Template_relevance Reaxys, Template_relevance Reaxys_biocatalysis model, leveraging a vast database of chemical reactions to predict feasible synthetic routes.
One-Step Synthesis Focus: Specifically designed for one-step synthesis, it provides concise and direct routes for your target compounds, streamlining the synthesis process.
Accurate Predictions: Utilizing the extensive PISTACHIO, BKMS_METABOLIC, PISTACHIO_RINGBREAKER, REAXYS, REAXYS_BIOCATALYSIS database, our tool offers high-accuracy predictions, reflecting the latest in chemical research and data.
Strategy Settings
Precursor scoring | Relevance Heuristic |
---|---|
Min. plausibility | 0.01 |
Model | Template_relevance |
Template Set | Pistachio/Bkms_metabolic/Pistachio_ringbreaker/Reaxys/Reaxys_biocatalysis |
Top-N result to add to graph | 6 |
Feasible Synthetic Routes
Disclaimer and Information on In-Vitro Research Products
Please be aware that all articles and product information presented on BenchChem are intended solely for informational purposes. The products available for purchase on BenchChem are specifically designed for in-vitro studies, which are conducted outside of living organisms. In-vitro studies, derived from the Latin term "in glass," involve experiments performed in controlled laboratory settings using cells or tissues. It is important to note that these products are not categorized as medicines or drugs, and they have not received approval from the FDA for the prevention, treatment, or cure of any medical condition, ailment, or disease. We must emphasize that any form of bodily introduction of these products into humans or animals is strictly prohibited by law. It is essential to adhere to these guidelines to ensure compliance with legal and ethical standards in research and experimentation.