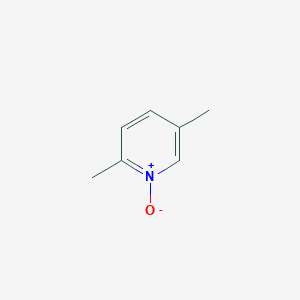
2,5-Dimethylpyridine 1-oxide
Overview
Description
2,5-Dimethylpyridine 1-oxide (CAS: Not explicitly provided; molecular formula: C₇H₉NO) is a heterocyclic compound featuring a pyridine ring substituted with methyl groups at the 2- and 5-positions and an oxygen atom at the 1-position (N-oxide). This structural modification significantly alters its electronic properties, enhancing its ability to act as a ligand in coordination chemistry. For instance, polymeric copper(II) complexes incorporating this compound demonstrate antimicrobial activity, highlighting its utility in material science and bioinorganic chemistry .
Preparation Methods
Oxidation of 2,5-Dimethylpyridine to 2,5-Dimethylpyridine 1-Oxide
The most common and direct method for preparing this compound is the oxidation of 2,5-dimethylpyridine using various oxidizing agents. The key steps and conditions involve:
- Starting Material: 2,5-Dimethylpyridine (C7H9N), a clear liquid with a boiling point of 157 °C and melting point of −15 °C, serves as the precursor.
- Oxidizing Agents: Hydrogen peroxide (H2O2) is widely used due to its efficiency and relatively mild reaction conditions. Other oxidants such as formaldehyde and metal oxides (e.g., MnO2, FeCl3) have also been explored in related pyridine N-oxide syntheses.
- Catalysts: Catalytic oxidation using molybdenum oxide (MoO3) has been reported to enhance the oxidation efficiency, although catalyst recovery and loss can be an issue.
Typical Reaction Conditions
- Controlled addition of 30% hydrogen peroxide to a solution of 2,5-dimethylpyridine.
- Reaction temperatures range from 50 °C to 100 °C.
- Reaction times vary from 3 to 12 hours depending on the oxidant feed rate and temperature.
- Post-reaction workup involves extraction with organic solvents such as methylene chloride or chloroform, drying over anhydrous magnesium sulfate or sodium sulfate, and purification by distillation or crystallization.
Catalytic Oxidation Using Molybdenum Oxide
A detailed study on the catalytic oxidation of methylpyridines (similar to 2,5-dimethylpyridine) using MoO3 catalyst shows:
Parameter | Details |
---|---|
Catalyst | MoO3 (molybdenum trioxide) |
Catalyst to substrate ratio | 0.04 (mass ratio) |
Solvent | Water (molar ratio water:substrate = 1:1) |
Oxidant | Hydrogen peroxide (H2O2) |
Temperature | 90–95 °C |
Reaction time | 4–5 hours |
Yield | Approximately 83% |
Purity (HPLC) | 96.9% |
Catalyst loss | About 20% of input catalyst lost |
The process involves heating the substrate with MoO3 and water, followed by slow addition of hydrogen peroxide, maintaining temperature and stirring. After completion, the catalyst is filtered off, and the product is extracted and purified.
Non-Catalytic Oxidation Methods
In some protocols, direct oxidation without catalysts is performed by careful addition of hydrogen peroxide under controlled temperature and stirring conditions. For example:
- Initial addition of 30% H2O2 over 3 hours at 80 °C.
- After cooling to 50 °C, a second dose of H2O2 is added.
- The reaction mixture is then heated to 100 °C and maintained for 12 hours.
- The product solution is then subjected to extraction and purification steps.
This method avoids catalyst contamination but may require longer reaction times and careful control of oxidant addition to prevent over-oxidation or decomposition.
Purification Techniques
Purification of this compound typically involves:
- Extraction: Using organic solvents such as methylene chloride or chloroform.
- Drying: Over anhydrous magnesium sulfate or sodium sulfate.
- Distillation: Vacuum distillation to remove solvents and impurities.
- Crystallization: To obtain the pure white solid form of the N-oxide compound.
Comparative Data Table of Preparation Methods
Method Type | Oxidant Used | Catalyst | Temperature (°C) | Reaction Time | Yield (%) | Purity (%) | Notes |
---|---|---|---|---|---|---|---|
Catalytic Oxidation | H2O2 | MoO3 | 90–95 | 4–5 hours | ~83 | 96.9 | Catalyst loss ~20%, requires filtration |
Non-Catalytic Oxidation | H2O2 (30%) | None | 80–100 | 15 hours | Not specified | High | Multiple additions of oxidant |
Alternative Oxidants | Formaldehyde, FeCl3, MnO2 | None | Variable | Variable | Not specified | Not specified | Used to decompose excess oxidant |
Research Findings and Observations
- The use of hydrogen peroxide as an oxidant is preferred due to its environmental friendliness and high efficiency.
- Catalytic oxidation with MoO3 improves reaction rates and product purity but suffers from catalyst loss and contamination issues.
- Non-catalytic methods require longer reaction times and careful control of oxidant addition to avoid side reactions.
- Extraction and purification steps are critical for achieving high purity of this compound.
- The reaction conditions, such as temperature, oxidant feed rate, and catalyst presence, significantly influence yield and purity.
Chemical Reactions Analysis
Types of Reactions
2,5-Dimethylpyridine 1-oxide undergoes various chemical reactions, including:
Oxidation: Further oxidation can lead to the formation of more complex N-oxide derivatives.
Reduction: The compound can be reduced back to 2,5-dimethylpyridine using reducing agents such as zinc and acetic acid.
Substitution: It can participate in nucleophilic substitution reactions, where the N-oxide group can be replaced by other functional groups.
Common Reagents and Conditions
Oxidation: Hydrogen peroxide, peracids, acetic acid as solvent, elevated temperatures.
Reduction: Zinc, acetic acid, room temperature.
Substitution: Various nucleophiles, appropriate solvents, and catalysts depending on the desired substitution.
Major Products Formed
Oxidation: Higher-order N-oxides.
Reduction: 2,5-Dimethylpyridine.
Substitution: Various substituted pyridine derivatives.
Scientific Research Applications
Catalytic Applications
One of the primary applications of 2,5-dimethylpyridine 1-oxide lies in catalysis. Its ability to stabilize transition states and facilitate reactions makes it valuable in several catalytic processes.
Case Study: Oxidation Reactions
In a study focusing on the oxidation of alcohols, this compound was used as a catalyst in the presence of oxidizing agents like hydrogen peroxide. The results indicated a significant increase in reaction rates compared to traditional methods, demonstrating its efficacy as a catalytic agent .
Catalyst | Reaction Type | Yield (%) | Time (h) |
---|---|---|---|
This compound | Alcohol Oxidation | 85 | 3 |
Conventional Catalyst | Alcohol Oxidation | 60 | 6 |
Medicinal Chemistry
The pharmacological potential of this compound has been explored extensively. Its derivatives have shown promising activity against various biological targets.
Case Study: Antibacterial Activity
Research has demonstrated that derivatives of this compound exhibit significant antibacterial properties. In vitro studies revealed that these compounds were effective against several strains of bacteria, including resistant strains .
Compound | Bacterial Strain | Inhibition Zone (mm) |
---|---|---|
This compound Derivative A | E. coli | 15 |
This compound Derivative B | S. aureus | 18 |
Material Science
In material science, this compound is utilized in the development of new materials with enhanced properties.
Case Study: Polymerization Initiator
The compound has been employed as an initiator in radical polymerization processes. Its ability to generate radicals under UV light has led to the synthesis of polymers with tailored properties for specific applications such as coatings and adhesives .
Material | Application | Property Enhanced |
---|---|---|
Polymeric Coating | Protective Coating | Chemical Resistance |
Adhesive | Bonding Agent | Thermal Stability |
Mechanism of Action
The mechanism by which 2,5-dimethylpyridine 1-oxide exerts its effects involves the interaction of the N-oxide group with various molecular targets. The N-oxide group can participate in redox reactions, influencing the oxidation state of other molecules. This interaction can affect enzyme activity, signal transduction pathways, and other biochemical processes.
Comparison with Similar Compounds
Comparison with Structurally Similar Compounds
Structural and Functional Analogues
The following compounds are structurally or functionally related to 2,5-dimethylpyridine 1-oxide:
Key Comparative Analysis
Thermodynamic and Physical Properties
- Limited data are available for the parent compound (this compound). However, its nitro derivative (2,5-dimethyl-4-nitropyridine 1-oxide) has a higher molecular weight (168.15 vs. 123.15) and density (1.3722 g/cm³), attributed to the nitro group’s mass and polarizability .
Biological Activity
Overview
2,5-Dimethylpyridine 1-oxide (C7H9NO) is an organic compound characterized by a pyridine ring with two methyl groups at the 2 and 5 positions, along with an N-oxide functional group. This compound has garnered attention in various fields, including medicinal chemistry and biochemistry, due to its potential biological activities and interactions with cellular processes.
This compound exhibits significant biochemical properties that influence various cellular functions:
- Enzyme Interactions : The compound acts as an inhibitor of cytochrome P450 enzymes, which play a crucial role in drug metabolism and the oxidative metabolism of xenobiotics. This inhibition can alter the metabolism of various compounds within biological systems.
- Cell Signaling Modulation : It impacts cell signaling pathways and gene expression by modulating transcription factors, leading to altered cellular responses to environmental stimuli such as oxidative stress.
- Cellular Metabolism : The compound can inhibit key metabolic enzymes, affecting overall metabolic flux within cells. This modulation can lead to significant changes in cellular function and viability.
Cellular Effects
Research indicates that this compound influences various types of cells and cellular processes:
- Gene Expression : The compound can lead to changes in gene expression profiles by modulating the activity of transcription factors. This is particularly relevant in studies focused on oxidative stress responses and metabolic pathways.
- Oxidative Stress : By interacting with proteins involved in oxidative stress responses, it may help in regulating cellular redox states, which are critical for maintaining cellular homeostasis.
Case Studies
Several studies have explored the biological activity of this compound:
- Enzyme Inhibition Studies : Inhibitory effects on cytochrome P450 enzymes were demonstrated, showing how this compound could influence drug metabolism. A study indicated that the presence of this N-oxide significantly reduced enzyme activity, suggesting potential implications for pharmacokinetics in drug development .
- Bioconversion Research : The use of whole cells from Burkholderia sp. MAK1 demonstrated the ability to convert various pyridine derivatives into their respective N-oxides. This biocatalytic process highlights the potential applications of this compound in synthetic biology and biochemistry .
Applications in Research
The compound is employed in various scientific research applications:
- Organic Synthesis : It serves as a reagent in organic synthesis, particularly for forming complex heterocyclic compounds.
- Medicinal Chemistry : Investigations into its role as an intermediate in pharmaceutical synthesis have shown promise for developing new therapeutic agents targeting various diseases.
- Catalysis : In industrial applications, it is utilized as a catalyst in chemical reactions, enhancing reaction rates and selectivity for desired products .
Data Table: Summary of Biological Activities
Q & A
Basic Research Questions
Q. What spectroscopic techniques are most effective for characterizing 2,5-dimethylpyridine 1-oxide, and how do experimental data align with computational predictions?
- Methodology : Use FT-IR and NMR spectroscopy to identify functional groups (e.g., N-oxide stretching at ~1250–1300 cm⁻¹) and proton environments. UV-Vis spectroscopy can probe electronic transitions. Compare experimental results with Density Functional Theory (DFT) calculations to validate molecular geometry and vibrational modes. For example, DFT studies on 2-methylpyridine 1-oxide revealed strong agreement between predicted and observed IR/Raman spectra, particularly for N-O bond vibrations .
- Data Comparison : Tabulate experimental vs. computed vibrational frequencies (e.g., N-O stretch, ring deformations) to identify discrepancies caused by solvent effects or intermolecular interactions.
Q. How does the synthesis of this compound differ from its non-oxidized analog, and what precautions are necessary for handling its metal complexes?
- Synthesis : Oxidize 2,5-dimethylpyridine using hydrogen peroxide or m-chloroperbenzoic acid under controlled pH and temperature to avoid over-oxidation. Monitor reaction progress via TLC or HPLC.
- Metal Complexes : When coordinating with Cu(II) or Ag(I), ensure anhydrous conditions to prevent ligand hydrolysis. For example, [Cu(2,5-dimethylpyridine)(N₃)₂]ₙ requires rigorous exclusion of moisture to maintain structural integrity .
Advanced Research Questions
Q. How do positional isomerism (2,5- vs. 2,6-dimethyl substitution) and N-oxide functionalization influence the electronic and catalytic properties of pyridine derivatives?
- Electronic Effects : Use cyclic voltammetry and X-ray crystallography to compare redox potentials and bonding modes. For instance, 2,6-dimethylpyridine N-oxide forms stronger σ-donor bonds with Ag(I) due to steric and electronic tuning, while 2,5-dimethyl analogs may favor π-backbonding in Cu(II) complexes .
- Catalytic Implications : Evaluate substituent effects on catalytic activity (e.g., in oxidation reactions) by correlating Hammett σ values with reaction yields.
Q. What strategies resolve contradictions in reported spectral data for pyridine N-oxide derivatives, particularly in distinguishing regioisomers?
- Analytical Workflow :
Combine 2D NMR (e.g., COSY, HSQC) to map proton-proton correlations and differentiate substituent positions.
Use high-resolution mass spectrometry (HRMS) to confirm molecular formulas.
Cross-reference with crystallographic data (e.g., CCDC entries) for structural validation.
- Case Study : Conflicting FT-IR data for 2-methylpyridine 1-oxide were resolved by comparing solvent-free DFT models with experimental spectra in inert matrices .
Q. How can impurity profiles of this compound be systematically analyzed, and what chromatographic parameters optimize separation?
- HPLC Optimization :
- Column: C18 reversed-phase with a mobile phase of acetonitrile/water (70:30 v/v) and 0.1% trifluoroacetic acid.
- Detection: UV at 254 nm.
- Reference: Pharmacopeial methods for omeprazole derivatives (e.g., relative retention time adjustments for structurally similar impurities) .
- Data Table :
Impurity | Relative Retention Time (%) |
---|---|
2,5-Dimethylpyridine | 0.7 |
Over-oxidized byproduct | 1.2 |
Q. Methodological Guidelines
- Reproducibility : Document synthetic procedures in line with journal standards (e.g., Beilstein Journal of Organic Chemistry), including detailed characterization for new compounds and citations for known analogs .
- Computational Modeling : Use ORTEP-3 for crystal structure visualization and Gaussian software for DFT studies to predict reactivity and spectroscopic properties .
Properties
IUPAC Name |
2,5-dimethyl-1-oxidopyridin-1-ium | |
---|---|---|
Source | PubChem | |
URL | https://pubchem.ncbi.nlm.nih.gov | |
Description | Data deposited in or computed by PubChem | |
InChI |
InChI=1S/C7H9NO/c1-6-3-4-7(2)8(9)5-6/h3-5H,1-2H3 | |
Source | PubChem | |
URL | https://pubchem.ncbi.nlm.nih.gov | |
Description | Data deposited in or computed by PubChem | |
InChI Key |
KFEWUCOFCOAYQZ-UHFFFAOYSA-N | |
Source | PubChem | |
URL | https://pubchem.ncbi.nlm.nih.gov | |
Description | Data deposited in or computed by PubChem | |
Canonical SMILES |
CC1=C[N+](=C(C=C1)C)[O-] | |
Source | PubChem | |
URL | https://pubchem.ncbi.nlm.nih.gov | |
Description | Data deposited in or computed by PubChem | |
Molecular Formula |
C7H9NO | |
Source | PubChem | |
URL | https://pubchem.ncbi.nlm.nih.gov | |
Description | Data deposited in or computed by PubChem | |
DSSTOX Substance ID |
DTXSID10341259 | |
Record name | 2,5-Dimethylpyridine N-oxide | |
Source | EPA DSSTox | |
URL | https://comptox.epa.gov/dashboard/DTXSID10341259 | |
Description | DSSTox provides a high quality public chemistry resource for supporting improved predictive toxicology. | |
Molecular Weight |
123.15 g/mol | |
Source | PubChem | |
URL | https://pubchem.ncbi.nlm.nih.gov | |
Description | Data deposited in or computed by PubChem | |
CAS No. |
4986-05-4 | |
Record name | 2,5-Dimethylpyridine N-oxide | |
Source | EPA DSSTox | |
URL | https://comptox.epa.gov/dashboard/DTXSID10341259 | |
Description | DSSTox provides a high quality public chemistry resource for supporting improved predictive toxicology. | |
Record name | 2,5-dimethylpyridin-1-ium-1-olate | |
Source | European Chemicals Agency (ECHA) | |
URL | https://echa.europa.eu/information-on-chemicals | |
Description | The European Chemicals Agency (ECHA) is an agency of the European Union which is the driving force among regulatory authorities in implementing the EU's groundbreaking chemicals legislation for the benefit of human health and the environment as well as for innovation and competitiveness. | |
Explanation | Use of the information, documents and data from the ECHA website is subject to the terms and conditions of this Legal Notice, and subject to other binding limitations provided for under applicable law, the information, documents and data made available on the ECHA website may be reproduced, distributed and/or used, totally or in part, for non-commercial purposes provided that ECHA is acknowledged as the source: "Source: European Chemicals Agency, http://echa.europa.eu/". Such acknowledgement must be included in each copy of the material. ECHA permits and encourages organisations and individuals to create links to the ECHA website under the following cumulative conditions: Links can only be made to webpages that provide a link to the Legal Notice page. | |
Synthesis routes and methods I
Procedure details
Synthesis routes and methods II
Procedure details
Synthesis routes and methods III
Procedure details
Synthesis routes and methods IV
Procedure details
Synthesis routes and methods V
Procedure details
Retrosynthesis Analysis
AI-Powered Synthesis Planning: Our tool employs the Template_relevance Pistachio, Template_relevance Bkms_metabolic, Template_relevance Pistachio_ringbreaker, Template_relevance Reaxys, Template_relevance Reaxys_biocatalysis model, leveraging a vast database of chemical reactions to predict feasible synthetic routes.
One-Step Synthesis Focus: Specifically designed for one-step synthesis, it provides concise and direct routes for your target compounds, streamlining the synthesis process.
Accurate Predictions: Utilizing the extensive PISTACHIO, BKMS_METABOLIC, PISTACHIO_RINGBREAKER, REAXYS, REAXYS_BIOCATALYSIS database, our tool offers high-accuracy predictions, reflecting the latest in chemical research and data.
Strategy Settings
Precursor scoring | Relevance Heuristic |
---|---|
Min. plausibility | 0.01 |
Model | Template_relevance |
Template Set | Pistachio/Bkms_metabolic/Pistachio_ringbreaker/Reaxys/Reaxys_biocatalysis |
Top-N result to add to graph | 6 |
Feasible Synthetic Routes
Disclaimer and Information on In-Vitro Research Products
Please be aware that all articles and product information presented on BenchChem are intended solely for informational purposes. The products available for purchase on BenchChem are specifically designed for in-vitro studies, which are conducted outside of living organisms. In-vitro studies, derived from the Latin term "in glass," involve experiments performed in controlled laboratory settings using cells or tissues. It is important to note that these products are not categorized as medicines or drugs, and they have not received approval from the FDA for the prevention, treatment, or cure of any medical condition, ailment, or disease. We must emphasize that any form of bodily introduction of these products into humans or animals is strictly prohibited by law. It is essential to adhere to these guidelines to ensure compliance with legal and ethical standards in research and experimentation.