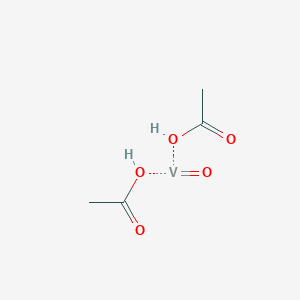
Vanadyl acetate
Overview
Description
Vanadyl acetate is a chemical compound that is used in scientific research for its potential therapeutic properties. It is a vanadium-containing compound that has been studied extensively for its ability to regulate glucose and lipid metabolism, as well as its antioxidant properties.
Scientific Research Applications
Anode Material for Lithium-Ion Batteries
Vanadyl Acetate has been used in the synthesis of one-dimensional nanobelts, which serve as a novel anode material for lithium-ion batteries . These nanobelts display outstanding electrochemical performance, including a highly stable reversible specific capacity and superior long-term cyclability .
Precursor for High Purity Compounds
It is used in the manufacturing of catalysts and nanoscale materials .
Catalyst for Epoxidation of Alkenes
Vanadium complexes, including Vanadyl Acetate, have been described as suitable catalysts for the epoxidation of small alkenes and allylic alcohols . This process has a wide range of valuable chemical applications, contributing to sustainable process chemistry .
Radiotracer in Positron Emission Tomography (PET)
Vanadyl Acetate has been used in the synthesis of potential radiotracers for positron emission tomography (PET) imaging . Specifically, Vanadium-48 (48 V) generated with a compact medical cyclotron has been used to label VO (acac) 2, a compound used in PET imaging for the detection of cancer .
Mechanism of Action
Target of Action
Vanadyl acetate primarily targets transition metal oxides (TMOs) . These TMOs are prospective anode materials for lithium-ion batteries (LIBs) due to their high theoretical specific capacity . Vanadyl acetate also targets protein tyrosine phosphatases (PTPase) , which are widely used as inhibitors in cell lysis buffers or immunoprecipitations of phosphotyrosyl proteins .
Mode of Action
Vanadyl acetate interacts with its targets by forming coordination complexes . For instance, it forms an organic-inorganic hybrid material known as vanadyl acetate nanobelts when coupled with lithium-ion conducting polymer ligands . This coupling is favorable for the dynamics of electrochemical processes . In the context of protein tyrosine phosphatases, vanadyl acetate acts as an inhibitor, affecting the dephosphorylation at the beta subunit of the insulin receptor .
Biochemical Pathways
Vanadyl acetate affects the electrochemical processes in lithium-ion batteries . It enhances the dynamics of these processes, leading to improved performance of the batteries . In biological systems, vanadyl acetate inhibits protein tyrosine phosphatases, impacting the phosphorylation pathways .
Pharmacokinetics
The pharmacokinetics of vanadyl acetate is complex due to its ability to form coordination complexes . Its absorption, distribution, metabolism, and excretion (ADME) properties are influenced by these complexes .
Result of Action
The result of vanadyl acetate’s action is the creation of a novel anode material for lithium-ion batteries . The vanadyl acetate nanobelt electrode displays outstanding electrochemical performance, including a highly stable reversible specific capacity, superior long-term cyclability, and attractive rate capability .
Action Environment
The action, efficacy, and stability of vanadyl acetate can be influenced by various environmental factors. For instance, the synthesis of vanadyl acetate nanobelts requires a specific temperature (200°C) and the presence of certain chemicals (vanadium pentoxide and lithium hydroxide in acetic acid or ethylene glycol) . In biological systems, the effects of vanadyl acetate can depend on the context of the cell types
properties
IUPAC Name |
acetic acid;oxovanadium | |
---|---|---|
Source | PubChem | |
URL | https://pubchem.ncbi.nlm.nih.gov | |
Description | Data deposited in or computed by PubChem | |
InChI |
InChI=1S/2C2H4O2.O.V/c2*1-2(3)4;;/h2*1H3,(H,3,4);; | |
Source | PubChem | |
URL | https://pubchem.ncbi.nlm.nih.gov | |
Description | Data deposited in or computed by PubChem | |
InChI Key |
HHCSCQJCUZGLHW-UHFFFAOYSA-N | |
Source | PubChem | |
URL | https://pubchem.ncbi.nlm.nih.gov | |
Description | Data deposited in or computed by PubChem | |
Canonical SMILES |
CC(=O)O.CC(=O)O.O=[V] | |
Source | PubChem | |
URL | https://pubchem.ncbi.nlm.nih.gov | |
Description | Data deposited in or computed by PubChem | |
Molecular Formula |
C4H8O5V | |
Source | PubChem | |
URL | https://pubchem.ncbi.nlm.nih.gov | |
Description | Data deposited in or computed by PubChem | |
DSSTOX Substance ID |
DTXSID001311115 | |
Record name | Bis(acetato-κO)oxovanadium | |
Source | EPA DSSTox | |
URL | https://comptox.epa.gov/dashboard/DTXSID001311115 | |
Description | DSSTox provides a high quality public chemistry resource for supporting improved predictive toxicology. | |
Molecular Weight |
187.04 g/mol | |
Source | PubChem | |
URL | https://pubchem.ncbi.nlm.nih.gov | |
Description | Data deposited in or computed by PubChem | |
CAS RN |
3473-84-5 | |
Record name | Bis(acetato-O)oxovanadium | |
Source | ChemIDplus | |
URL | https://pubchem.ncbi.nlm.nih.gov/substance/?source=chemidplus&sourceid=0003473845 | |
Description | ChemIDplus is a free, web search system that provides access to the structure and nomenclature authority files used for the identification of chemical substances cited in National Library of Medicine (NLM) databases, including the TOXNET system. | |
Record name | Bis(acetato-κO)oxovanadium | |
Source | EPA DSSTox | |
URL | https://comptox.epa.gov/dashboard/DTXSID001311115 | |
Description | DSSTox provides a high quality public chemistry resource for supporting improved predictive toxicology. | |
Record name | Bis(acetato-O)oxovanadium | |
Source | European Chemicals Agency (ECHA) | |
URL | https://echa.europa.eu/substance-information/-/substanceinfo/100.020.406 | |
Description | The European Chemicals Agency (ECHA) is an agency of the European Union which is the driving force among regulatory authorities in implementing the EU's groundbreaking chemicals legislation for the benefit of human health and the environment as well as for innovation and competitiveness. | |
Explanation | Use of the information, documents and data from the ECHA website is subject to the terms and conditions of this Legal Notice, and subject to other binding limitations provided for under applicable law, the information, documents and data made available on the ECHA website may be reproduced, distributed and/or used, totally or in part, for non-commercial purposes provided that ECHA is acknowledged as the source: "Source: European Chemicals Agency, http://echa.europa.eu/". Such acknowledgement must be included in each copy of the material. ECHA permits and encourages organisations and individuals to create links to the ECHA website under the following cumulative conditions: Links can only be made to webpages that provide a link to the Legal Notice page. | |
Retrosynthesis Analysis
AI-Powered Synthesis Planning: Our tool employs the Template_relevance Pistachio, Template_relevance Bkms_metabolic, Template_relevance Pistachio_ringbreaker, Template_relevance Reaxys, Template_relevance Reaxys_biocatalysis model, leveraging a vast database of chemical reactions to predict feasible synthetic routes.
One-Step Synthesis Focus: Specifically designed for one-step synthesis, it provides concise and direct routes for your target compounds, streamlining the synthesis process.
Accurate Predictions: Utilizing the extensive PISTACHIO, BKMS_METABOLIC, PISTACHIO_RINGBREAKER, REAXYS, REAXYS_BIOCATALYSIS database, our tool offers high-accuracy predictions, reflecting the latest in chemical research and data.
Strategy Settings
Precursor scoring | Relevance Heuristic |
---|---|
Min. plausibility | 0.01 |
Model | Template_relevance |
Template Set | Pistachio/Bkms_metabolic/Pistachio_ringbreaker/Reaxys/Reaxys_biocatalysis |
Top-N result to add to graph | 6 |
Feasible Synthetic Routes
Q & A
Q1: What is the structure of vanadyl acetate and how does it influence its magnetic properties?
A1: Vanadyl acetate (VO(CH3COO)2) features vanadium atoms in an octahedral coordination geometry. [] These VO6 octahedra are linked together through vanadyl groups and bridging acetate groups, forming a one-dimensional chain structure. [] This arrangement leads to magnetic interactions between the vanadium atoms along the chain, resulting in antiferromagnetic behavior. []
Q2: How does the synthesis method affect the morphology and application of vanadyl acetate?
A2: Vanadyl acetate nanobelts can be synthesized via a facile solvothermal method. [] This morphology is particularly interesting for lithium-ion battery applications, as it exhibits excellent electrochemical performance as an anode material. []
Q3: Can you elaborate on the electrochemical properties of vanadyl acetate nanobelts for lithium-ion batteries?
A3: Vanadyl acetate nanobelts demonstrate a high reversible specific capacity (around 1065 mA h g-1 at 200 mA g-1) and impressive long-term cyclability, retaining a capacity of approximately 477 mA h g-1 at 2 A g-1 even after 500 cycles. [] Additionally, they exhibit attractive rate capability. []
Q4: What analytical techniques are used to characterize the structural changes of vanadyl acetate during electrochemical cycling?
A4: In situ XRD analysis is a powerful tool for investigating the structural variations of vanadyl acetate electrodes during lithium-ion insertion/extraction processes. [] The periodic evolution of the in situ XRD patterns provides evidence of excellent reversibility for Li+ ion insertion/extraction within the vanadyl acetate nanobelt electrode. []
Disclaimer and Information on In-Vitro Research Products
Please be aware that all articles and product information presented on BenchChem are intended solely for informational purposes. The products available for purchase on BenchChem are specifically designed for in-vitro studies, which are conducted outside of living organisms. In-vitro studies, derived from the Latin term "in glass," involve experiments performed in controlled laboratory settings using cells or tissues. It is important to note that these products are not categorized as medicines or drugs, and they have not received approval from the FDA for the prevention, treatment, or cure of any medical condition, ailment, or disease. We must emphasize that any form of bodily introduction of these products into humans or animals is strictly prohibited by law. It is essential to adhere to these guidelines to ensure compliance with legal and ethical standards in research and experimentation.