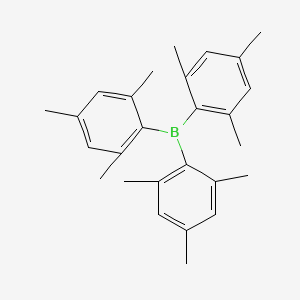
Trimesitylborane
Overview
Description
Trimesitylborane, also known as tris(2,4,6-trimethylphenyl)borane, is a chemical compound with the molecular formula C27H33B. It is a triarylborane, where the boron atom is bonded to three mesityl groups (2,4,6-trimethylphenyl groups). This compound is known for its stability and unique reactivity, making it a valuable reagent in various chemical reactions .
Mechanism of Action
Target of Action
Trimesitylborane, also known as Tris(2,4,6-trimethylphenyl)borane, is primarily used as a reducing agent . Its primary targets are molecules that can be reduced or molecules that can form adducts with boron .
Mode of Action
This compound interacts with its targets through a process known as reduction. It donates electrons to other molecules, thereby reducing them . In the case of anion receptors, this compound can form adducts with small anions .
Biochemical Pathways
It is known to be involved in the synthesis of sandwich complexes of this compound and fluoroborate and cyanoborate derivatives . It also plays a role in the Suzuki-Miyaura coupling conditions .
Pharmacokinetics
It is known that this compound is a solid compound with a melting point of 193-195 °c (lit) and is typically stored at 2-8°C .
Result of Action
The result of this compound’s action depends on the specific reaction it is involved in. For example, in the synthesis of sandwich complexes, it reacts with both fluoride and cyanide anions in THF or MeOH to afford the corresponding zwitterionic fluoro - (1-F) and cyano - borate (1-CN) species .
Action Environment
The action of this compound can be influenced by environmental factors such as temperature and the presence of other chemicals. For instance, compounds 1 and 2 synthesized from this compound are hydrolyzed on prolonged exposure to air . Additionally, the reaction of this compound with fluoride anions results in boryl-carbo-fluorane bond cleavage .
Biochemical Analysis
Biochemical Properties
Trimesitylborane plays a significant role in biochemical reactions due to its ability to act as a Lewis acid. It interacts with various enzymes, proteins, and other biomolecules. For instance, this compound has been used in the synthesis of sandwich complexes with fluoroborate and cyanoborate derivatives . These interactions are crucial for understanding the reactivity and stability of this compound in different biochemical environments.
Cellular Effects
The effects of this compound on various types of cells and cellular processes are profound. It influences cell function by affecting cell signaling pathways, gene expression, and cellular metabolism. This compound’s ability to interact with biomolecules can lead to changes in cellular activities, which are essential for understanding its potential therapeutic applications and toxicity .
Molecular Mechanism
At the molecular level, this compound exerts its effects through binding interactions with biomolecules. It can inhibit or activate enzymes, leading to changes in gene expression. The compound’s Lewis acidic nature allows it to form stable complexes with various anions, which can influence biochemical pathways and cellular functions .
Temporal Effects in Laboratory Settings
In laboratory settings, the effects of this compound change over time. The compound’s stability and degradation are critical factors that influence its long-term effects on cellular function. Studies have shown that this compound remains stable under specific conditions, but its reactivity can vary depending on the experimental setup .
Dosage Effects in Animal Models
The effects of this compound vary with different dosages in animal models. At lower doses, the compound may exhibit beneficial effects, while higher doses could lead to toxicity or adverse effects. Understanding the threshold effects and safe dosage ranges is essential for potential therapeutic applications of this compound .
Metabolic Pathways
This compound is involved in various metabolic pathways, interacting with enzymes and cofactors. These interactions can affect metabolic flux and metabolite levels, providing insights into the compound’s role in cellular metabolism. The study of these pathways is crucial for understanding the biochemical properties of this compound .
Transport and Distribution
Within cells and tissues, this compound is transported and distributed through interactions with transporters and binding proteins. These interactions influence the compound’s localization and accumulation, affecting its overall activity and function in biochemical processes .
Subcellular Localization
The subcellular localization of this compound is determined by targeting signals and post-translational modifications. These factors direct the compound to specific compartments or organelles, influencing its activity and function. Understanding the subcellular localization of this compound is essential for elucidating its biochemical roles .
Preparation Methods
Synthetic Routes and Reaction Conditions: Trimesitylborane can be synthesized through the reaction of boron trichloride with mesityl magnesium bromide in an ether solvent. The reaction typically proceeds as follows:
BCl3+3C6H2(CH3)3MgBr→B(C6H2(CH3)3)3+3MgBrCl
The reaction is carried out under an inert atmosphere to prevent the hydrolysis of boron trichloride .
Industrial Production Methods: While there is limited information on the large-scale industrial production of this compound, the laboratory synthesis methods can be scaled up with appropriate modifications to reaction conditions and purification processes .
Chemical Reactions Analysis
Types of Reactions: Trimesitylborane undergoes various types of chemical reactions, including:
Oxidation: this compound can be oxidized to form this compound oxide.
Reduction: It can be reduced to form borohydride derivatives.
Substitution: The mesityl groups can be substituted with other aryl or alkyl groups under specific conditions.
Common Reagents and Conditions:
Oxidation: Common oxidizing agents include hydrogen peroxide and oxygen.
Reduction: Reducing agents such as lithium aluminum hydride can be used.
Substitution: Reagents like organolithium or organomagnesium compounds are commonly used.
Major Products:
Oxidation: this compound oxide.
Reduction: Borohydride derivatives.
Substitution: Various substituted boranes depending on the reagents used.
Scientific Research Applications
Trimesitylborane has several applications in scientific research, including:
Chemistry: It is used as a reagent in the synthesis of complex organic molecules and as a catalyst in various organic reactions.
Biology: this compound derivatives are studied for their potential use in biological imaging and as probes for detecting specific biomolecules.
Medicine: Research is ongoing to explore the use of this compound derivatives in drug delivery systems and as therapeutic agents.
Industry: It is used in the production of advanced materials, including polymers and electronic components
Comparison with Similar Compounds
Triphenylborane: Similar to trimesitylborane but with phenyl groups instead of mesityl groups.
Tris(pentafluorophenyl)borane: Contains pentafluorophenyl groups, making it more electron-deficient and reactive.
Dimesitylboron fluoride: Contains two mesityl groups and one fluoride, offering different reactivity and stability
Uniqueness of this compound: this compound is unique due to the presence of three mesityl groups, which provide both steric protection and electronic effects that enhance its stability and reactivity. This makes it a valuable reagent in various chemical reactions and applications .
Properties
IUPAC Name |
tris(2,4,6-trimethylphenyl)borane | |
---|---|---|
Source | PubChem | |
URL | https://pubchem.ncbi.nlm.nih.gov | |
Description | Data deposited in or computed by PubChem | |
InChI |
InChI=1S/C27H33B/c1-16-10-19(4)25(20(5)11-16)28(26-21(6)12-17(2)13-22(26)7)27-23(8)14-18(3)15-24(27)9/h10-15H,1-9H3 | |
Source | PubChem | |
URL | https://pubchem.ncbi.nlm.nih.gov | |
Description | Data deposited in or computed by PubChem | |
InChI Key |
FXFWLXFJBVILBE-UHFFFAOYSA-N | |
Source | PubChem | |
URL | https://pubchem.ncbi.nlm.nih.gov | |
Description | Data deposited in or computed by PubChem | |
Canonical SMILES |
B(C1=C(C=C(C=C1C)C)C)(C2=C(C=C(C=C2C)C)C)C3=C(C=C(C=C3C)C)C | |
Source | PubChem | |
URL | https://pubchem.ncbi.nlm.nih.gov | |
Description | Data deposited in or computed by PubChem | |
Molecular Formula |
C27H33B | |
Source | PubChem | |
URL | https://pubchem.ncbi.nlm.nih.gov | |
Description | Data deposited in or computed by PubChem | |
DSSTOX Substance ID |
DTXSID80302271 | |
Record name | Trimesitylborane | |
Source | EPA DSSTox | |
URL | https://comptox.epa.gov/dashboard/DTXSID80302271 | |
Description | DSSTox provides a high quality public chemistry resource for supporting improved predictive toxicology. | |
Molecular Weight |
368.4 g/mol | |
Source | PubChem | |
URL | https://pubchem.ncbi.nlm.nih.gov | |
Description | Data deposited in or computed by PubChem | |
CAS No. |
7297-95-2 | |
Record name | Trimesitylborane | |
Source | DTP/NCI | |
URL | https://dtp.cancer.gov/dtpstandard/servlet/dwindex?searchtype=NSC&outputformat=html&searchlist=149907 | |
Description | The NCI Development Therapeutics Program (DTP) provides services and resources to the academic and private-sector research communities worldwide to facilitate the discovery and development of new cancer therapeutic agents. | |
Explanation | Unless otherwise indicated, all text within NCI products is free of copyright and may be reused without our permission. Credit the National Cancer Institute as the source. | |
Record name | Trimesitylborane | |
Source | EPA DSSTox | |
URL | https://comptox.epa.gov/dashboard/DTXSID80302271 | |
Description | DSSTox provides a high quality public chemistry resource for supporting improved predictive toxicology. | |
Retrosynthesis Analysis
AI-Powered Synthesis Planning: Our tool employs the Template_relevance Pistachio, Template_relevance Bkms_metabolic, Template_relevance Pistachio_ringbreaker, Template_relevance Reaxys, Template_relevance Reaxys_biocatalysis model, leveraging a vast database of chemical reactions to predict feasible synthetic routes.
One-Step Synthesis Focus: Specifically designed for one-step synthesis, it provides concise and direct routes for your target compounds, streamlining the synthesis process.
Accurate Predictions: Utilizing the extensive PISTACHIO, BKMS_METABOLIC, PISTACHIO_RINGBREAKER, REAXYS, REAXYS_BIOCATALYSIS database, our tool offers high-accuracy predictions, reflecting the latest in chemical research and data.
Strategy Settings
Precursor scoring | Relevance Heuristic |
---|---|
Min. plausibility | 0.01 |
Model | Template_relevance |
Template Set | Pistachio/Bkms_metabolic/Pistachio_ringbreaker/Reaxys/Reaxys_biocatalysis |
Top-N result to add to graph | 6 |
Feasible Synthetic Routes
Disclaimer and Information on In-Vitro Research Products
Please be aware that all articles and product information presented on BenchChem are intended solely for informational purposes. The products available for purchase on BenchChem are specifically designed for in-vitro studies, which are conducted outside of living organisms. In-vitro studies, derived from the Latin term "in glass," involve experiments performed in controlled laboratory settings using cells or tissues. It is important to note that these products are not categorized as medicines or drugs, and they have not received approval from the FDA for the prevention, treatment, or cure of any medical condition, ailment, or disease. We must emphasize that any form of bodily introduction of these products into humans or animals is strictly prohibited by law. It is essential to adhere to these guidelines to ensure compliance with legal and ethical standards in research and experimentation.