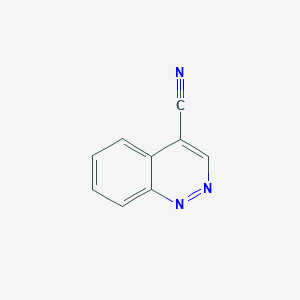
cinnoline-4-carbonitrile
Overview
Description
cinnoline-4-carbonitrile is a heterocyclic organic compound with the chemical formula C9H5N3. It is a yellow crystalline solid that finds applications in various fields such as medical research, environmental research, and industrial research. The compound is known for its unique structure, which includes a cinnoline ring fused with a nitrile group, making it a valuable intermediate in organic synthesis.
Mechanism of Action
Target of Action
Cinnoline derivatives are known to have a broad range of biological properties and are used as synthetic intermediates, potential drug candidates, and chemical probes .
Mode of Action
It’s known that a nitrile function in the 4-position of the cinnoline system can be readily displaced by various nucleophiles . This suggests that cinnoline-4-carbonitrile may interact with its targets through nucleophilic substitution reactions.
Biochemical Pathways
They are indispensable moieties to treat infectious diseases , indicating their involvement in various biochemical pathways related to immune response and pathogen defense.
Pharmacokinetics
The development of cinnoline-based molecules is known to contribute significantly to the identification of lead compounds with optimized pharmacodynamic and pharmacokinetic properties .
Result of Action
Cinnoline derivatives are known to exhibit a broad spectrum of pharmacological activities such as antibacterial, antifungal, antimalarial, anti-inflammatory, analgesic, anxiolytic, and antitumor activities .
Action Environment
It’s worth noting that the synthesis of cinnoline derivatives can be achieved via sustainable synthetic approaches , suggesting that environmental factors may play a role in the synthesis and stability of these compounds.
Preparation Methods
Synthetic Routes and Reaction Conditions: The synthesis of cinnoline-4-carbonitrile typically involves the reaction of cinnoline derivatives with nitrile-containing reagents. One common method is the reaction of 4-chlorocinnoline with sodium cyanide in the presence of a suitable solvent like dimethyl sulfoxide (DMSO). The reaction is carried out under reflux conditions to yield this compound .
Industrial Production Methods: Industrial production of this compound follows similar synthetic routes but on a larger scale. The process involves the use of high-purity reagents and controlled reaction conditions to ensure a high yield and purity of the final product. The reaction is typically carried out in large reactors with continuous monitoring of temperature, pressure, and reaction time to optimize the production process .
Chemical Reactions Analysis
Types of Reactions: cinnoline-4-carbonitrile undergoes various chemical reactions, including:
Oxidation: The compound can be oxidized using strong oxidizing agents like potassium permanganate or chromium trioxide to form corresponding oxidized derivatives.
Reduction: Reduction of this compound can be achieved using reducing agents such as lithium aluminum hydride or sodium borohydride, leading to the formation of amine derivatives.
Substitution: The nitrile group in this compound can undergo nucleophilic substitution reactions with various nucleophiles, resulting in the formation of substituted cinnoline derivatives.
Common Reagents and Conditions:
Oxidation: Potassium permanganate in acidic or neutral medium.
Reduction: Lithium aluminum hydride in anhydrous ether.
Substitution: Sodium cyanide in DMSO under reflux conditions.
Major Products Formed:
Oxidation: Oxidized cinnoline derivatives.
Reduction: Amine derivatives of cinnoline.
Substitution: Substituted cinnoline derivatives.
Scientific Research Applications
Medicinal Chemistry Applications
Cinnoline-4-carbonitrile and its derivatives have shown promising biological activities, making them valuable in drug discovery.
Anticancer Activity
Recent studies have highlighted the potential of cinnoline derivatives as inhibitors of tubulin polymerization, which is crucial in cancer cell proliferation. For instance, novel cinnolone derivatives synthesized via a three-step synthetic protocol exhibited significant inhibitory activity with a computational binding affinity of against tubulin . This suggests that this compound could serve as a lead compound for developing new anticancer agents.
Inhibition of Human Neutrophil Elastase (HNE)
Cinnoline derivatives have been identified as reversible competitive inhibitors of human neutrophil elastase (HNE), an important target in inflammatory diseases. The most potent compound from this class demonstrated an IC50 value of . Molecular docking studies confirmed the interaction between these compounds and the HNE binding site, indicating their potential therapeutic utility in treating conditions associated with excessive elastase activity.
Fluorogenic Probes
A novel fluorogenic probe based on the transformation of 4-azidocinnoline to cinnoline-4-amine was developed, demonstrating strong fluorescence in polar solvents like water. This probe was tested on HepG2 liver cancer cells, showcasing its applicability in cellular imaging and monitoring intracellular processes . The ability to visualize cellular events makes this compound a candidate for developing advanced diagnostic tools.
Antimicrobial Properties
Cinnoline derivatives have also been explored for their antimicrobial properties. A study reported that certain cinnoline compounds exhibited antibacterial activity against both Gram-negative and Gram-positive bacterial strains, including Pseudomonas aeruginosa and Staphylococcus aureus. This highlights their potential as therapeutic agents in combating bacterial infections.
Material Science Applications
The unique properties of this compound extend beyond biological applications into material science. For example:
Green Synthesis
A green synthesis approach utilizing natural microcapsules has been developed for producing cinnoline derivatives, which demonstrated promising optical properties and antibacterial activity . This method not only enhances the efficiency of synthesis but also minimizes environmental impact.
Summary of Findings
The following table summarizes key findings related to the applications of this compound:
Comparison with Similar Compounds
4-Isoquinolinecarbonitrile: Similar in structure but with an isoquinoline ring instead of a cinnoline ring.
4-Quinoxalinecarbonitrile: Contains a quinoxaline ring fused with a nitrile group.
4-(Methylsulfonyl)cinnoline: A derivative of cinnoline with a methylsulfonyl group.
Uniqueness: cinnoline-4-carbonitrile is unique due to its specific cinnoline ring structure fused with a nitrile group, which imparts distinct chemical and biological properties. Its ability to undergo various chemical reactions and its wide range of applications in different fields make it a valuable compound for research and industrial purposes .
Biological Activity
Cinnoline-4-carbonitrile is a heterocyclic compound that has garnered attention for its diverse biological activities. This article delves into its pharmacological properties, synthesis, and potential applications in medicine, supported by data tables and case studies.
Chemical Structure and Synthesis
This compound features a cinnoline ring with a cyano group at the 4-position. Its synthesis often involves various methods, including cyclocondensation reactions and microwave-assisted techniques. Recent studies have demonstrated efficient synthetic routes yielding high purity and biological activity.
Biological Activities
This compound and its derivatives exhibit a range of biological activities:
- Antimicrobial Activity : Research indicates that cinnoline derivatives possess significant antibacterial properties against both Gram-positive and Gram-negative bacteria. For instance, a study highlighted the effectiveness of certain cinnoline derivatives against Escherichia coli and Staphylococcus aureus, showcasing their potential as antimicrobial agents .
- Anticancer Properties : Several studies have reported the anticancer activity of cinnoline derivatives. A review noted that specific compounds demonstrated cytotoxic effects on various cancer cell lines, suggesting mechanisms involving apoptosis induction and cell cycle arrest .
- Anti-inflammatory Effects : Cinnoline derivatives have shown promise in reducing inflammation. In experimental models, compounds such as 3-acetyl-6-fluoro-cinnoline demonstrated significant anti-inflammatory activity, with percentage inhibition rates comparable to standard anti-inflammatory drugs .
Case Studies
-
Antibacterial Efficacy :
- A study tested a series of cinnoline derivatives against multiple bacterial strains, finding that compounds with halogen substitutions exhibited enhanced antibacterial activity.
- The results indicated that 7-chloro-6-fluoro-cinnoline derivatives had minimum inhibitory concentrations (MICs) lower than those of conventional antibiotics .
- Antitumor Activity :
- Anti-inflammatory Studies :
Table 1: Antibacterial Activity of Cinnoline Derivatives
Compound | Bacterial Strain | MIC (µg/mL) |
---|---|---|
7-Chloro-6-fluoro-cinnoline | E. coli | 12 |
7-Bromo-cinnoline | S. aureus | 8 |
3-Acetyl-cinnoline | Pseudomonas aeruginosa | 10 |
Table 2: Anticancer Activity (IC50 Values)
Compound | Cell Line | IC50 (µM) |
---|---|---|
This compound | HeLa | 15 |
Cinnoline derivative A | MCF-7 | 20 |
Cinnoline derivative B | A549 | 18 |
Properties
IUPAC Name |
cinnoline-4-carbonitrile | |
---|---|---|
Source | PubChem | |
URL | https://pubchem.ncbi.nlm.nih.gov | |
Description | Data deposited in or computed by PubChem | |
InChI |
InChI=1S/C9H5N3/c10-5-7-6-11-12-9-4-2-1-3-8(7)9/h1-4,6H | |
Source | PubChem | |
URL | https://pubchem.ncbi.nlm.nih.gov | |
Description | Data deposited in or computed by PubChem | |
InChI Key |
SKALQBBFRXNOBD-UHFFFAOYSA-N | |
Source | PubChem | |
URL | https://pubchem.ncbi.nlm.nih.gov | |
Description | Data deposited in or computed by PubChem | |
Canonical SMILES |
C1=CC=C2C(=C1)C(=CN=N2)C#N | |
Source | PubChem | |
URL | https://pubchem.ncbi.nlm.nih.gov | |
Description | Data deposited in or computed by PubChem | |
Molecular Formula |
C9H5N3 | |
Source | PubChem | |
URL | https://pubchem.ncbi.nlm.nih.gov | |
Description | Data deposited in or computed by PubChem | |
DSSTOX Substance ID |
DTXSID80343927 | |
Record name | 4-Cinnolinecarbonitrile | |
Source | EPA DSSTox | |
URL | https://comptox.epa.gov/dashboard/DTXSID80343927 | |
Description | DSSTox provides a high quality public chemistry resource for supporting improved predictive toxicology. | |
Molecular Weight |
155.16 g/mol | |
Source | PubChem | |
URL | https://pubchem.ncbi.nlm.nih.gov | |
Description | Data deposited in or computed by PubChem | |
CAS No. |
16470-90-9 | |
Record name | 4-Cinnolinecarbonitrile | |
Source | EPA DSSTox | |
URL | https://comptox.epa.gov/dashboard/DTXSID80343927 | |
Description | DSSTox provides a high quality public chemistry resource for supporting improved predictive toxicology. | |
Synthesis routes and methods
Procedure details
Retrosynthesis Analysis
AI-Powered Synthesis Planning: Our tool employs the Template_relevance Pistachio, Template_relevance Bkms_metabolic, Template_relevance Pistachio_ringbreaker, Template_relevance Reaxys, Template_relevance Reaxys_biocatalysis model, leveraging a vast database of chemical reactions to predict feasible synthetic routes.
One-Step Synthesis Focus: Specifically designed for one-step synthesis, it provides concise and direct routes for your target compounds, streamlining the synthesis process.
Accurate Predictions: Utilizing the extensive PISTACHIO, BKMS_METABOLIC, PISTACHIO_RINGBREAKER, REAXYS, REAXYS_BIOCATALYSIS database, our tool offers high-accuracy predictions, reflecting the latest in chemical research and data.
Strategy Settings
Precursor scoring | Relevance Heuristic |
---|---|
Min. plausibility | 0.01 |
Model | Template_relevance |
Template Set | Pistachio/Bkms_metabolic/Pistachio_ringbreaker/Reaxys/Reaxys_biocatalysis |
Top-N result to add to graph | 6 |
Feasible Synthetic Routes
Disclaimer and Information on In-Vitro Research Products
Please be aware that all articles and product information presented on BenchChem are intended solely for informational purposes. The products available for purchase on BenchChem are specifically designed for in-vitro studies, which are conducted outside of living organisms. In-vitro studies, derived from the Latin term "in glass," involve experiments performed in controlled laboratory settings using cells or tissues. It is important to note that these products are not categorized as medicines or drugs, and they have not received approval from the FDA for the prevention, treatment, or cure of any medical condition, ailment, or disease. We must emphasize that any form of bodily introduction of these products into humans or animals is strictly prohibited by law. It is essential to adhere to these guidelines to ensure compliance with legal and ethical standards in research and experimentation.