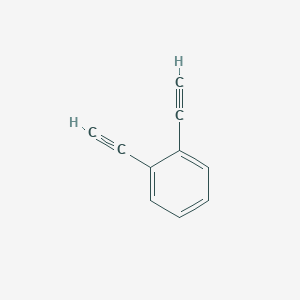
1,2-Diethynylbenzene
Overview
Description
1,2-Diethynylbenzene is an organic compound with the molecular formula C₁₀H₆. It consists of a benzene ring substituted with two ethynyl groups at the 1 and 2 positions.
Preparation Methods
Synthetic Routes and Reaction Conditions: 1,2-Diethynylbenzene can be synthesized through the oxidative coupling of this compound derivatives. This method involves the use of oxidative agents to couple the ethynyl groups, forming the desired compound . The reaction conditions typically include the use of a solvent such as tetrahydrofuran and a catalyst like copper(I) chloride.
Industrial Production Methods: While specific industrial production methods for this compound are not extensively documented, the general approach involves large-scale oxidative coupling reactions. The process may be optimized for higher yields and purity by adjusting reaction parameters such as temperature, pressure, and catalyst concentration.
Chemical Reactions Analysis
Types of Reactions: 1,2-Diethynylbenzene undergoes various types of chemical reactions, including:
Reduction: Reduction reactions can convert the ethynyl groups to ethylene groups, altering the compound’s reactivity and properties.
Common Reagents and Conditions:
Oxidation: Reagents such as iodine or other halogens can be used under controlled conditions to achieve oxidation.
Reduction: Hydrogenation reactions using catalysts like palladium on carbon can reduce the ethynyl groups.
Substitution: Electrophilic reagents such as bromine or nitronium ions can be used in the presence of a Lewis acid catalyst.
Major Products Formed:
Oxidation: Diacetylene macrocycles and other polymeric structures.
Reduction: Ethylene-substituted benzene derivatives.
Substitution: Various substituted benzene derivatives depending on the electrophile used.
Scientific Research Applications
1,2-Diethynylbenzene has several scientific research applications, including:
Materials Science: It is used as a precursor for the synthesis of novel conjugated organic polymers, which have applications in electronics and photonics.
Chemistry: The compound is utilized in the study of aromatic substitution reactions and the development of new synthetic methodologies.
Mechanism of Action
The mechanism of action of 1,2-diethynylbenzene primarily involves its ability to undergo oxidative coupling and polymerization reactions. The ethynyl groups can participate in various chemical transformations, leading to the formation of complex structures. The molecular targets and pathways involved include the interaction of the ethynyl groups with oxidative agents and catalysts, facilitating the formation of new bonds and polymeric structures .
Comparison with Similar Compounds
- 1,3-Diethynylbenzene
- 1,4-Diethynylbenzene
- Diethynylbenzene dianion
Comparison: 1,2-Diethynylbenzene is unique due to the specific positioning of the ethynyl groups on the benzene ring, which influences its reactivity and the types of reactions it can undergo. Compared to 1,3-diethynylbenzene and 1,4-diethynylbenzene, the 1,2-isomer exhibits different electronic and steric properties, leading to distinct chemical behavior and applications .
Biological Activity
1,2-Diethynylbenzene, a compound characterized by its unique structure featuring two ethynyl groups attached to a benzene ring, has garnered interest in various fields due to its biological activities and potential applications in medicinal chemistry. This article explores the biological activity of this compound, highlighting its mechanisms of action, relevant case studies, and research findings.
Chemical Structure and Properties
This compound (C10H6) is an aromatic compound that can undergo various chemical reactions due to the presence of the ethynyl groups. Its structure allows it to participate in cycloaddition reactions and other transformations that are relevant for biological applications.
1. Inhibition of Enzymatic Activity
Recent studies have demonstrated that this compound can act as a mechanism-based inactivator of certain enzymes. For instance, it has been shown to inhibit phenol hydroxylase in Pseudomonas sp. CF600, which is crucial for phenol degradation. The compound's ability to covalently modify the enzyme's active site leads to a decrease in enzymatic activity, suggesting its potential use as an inhibitor in bioremediation processes .
2. Antitumor Activity
Research has indicated that derivatives of this compound exhibit cytotoxic properties against various cancer cell lines. The compound's reactivity allows it to form reactive intermediates that can interact with cellular macromolecules, potentially leading to apoptosis in tumor cells. Studies on related enediynes have highlighted their potent antitumor activity, suggesting that this compound may share similar properties .
Case Study 1: Enzyme Inhibition
A study utilized activity-based fluorescent labeling to explore the effects of this compound on Pseudomonas sp. CF600. The results showed that treatment with the compound led to specific labeling of polypeptides associated with phenol hydroxylase, demonstrating its role as an effective inhibitor .
Case Study 2: Antitumor Potential
In another investigation focused on the synthesis of enediyne compounds derived from this compound, researchers found that these derivatives exhibited significant cytotoxicity against several cancer cell lines. The mechanism was attributed to their ability to generate reactive oxygen species (ROS), which can induce oxidative stress and lead to cell death .
Comparative Table of Biological Activities
Properties
IUPAC Name |
1,2-diethynylbenzene | |
---|---|---|
Source | PubChem | |
URL | https://pubchem.ncbi.nlm.nih.gov | |
Description | Data deposited in or computed by PubChem | |
InChI |
InChI=1S/C10H6/c1-3-9-7-5-6-8-10(9)4-2/h1-2,5-8H | |
Source | PubChem | |
URL | https://pubchem.ncbi.nlm.nih.gov | |
Description | Data deposited in or computed by PubChem | |
InChI Key |
CBYDUPRWILCUIC-UHFFFAOYSA-N | |
Source | PubChem | |
URL | https://pubchem.ncbi.nlm.nih.gov | |
Description | Data deposited in or computed by PubChem | |
Canonical SMILES |
C#CC1=CC=CC=C1C#C | |
Source | PubChem | |
URL | https://pubchem.ncbi.nlm.nih.gov | |
Description | Data deposited in or computed by PubChem | |
Molecular Formula |
C10H6 | |
Source | PubChem | |
URL | https://pubchem.ncbi.nlm.nih.gov | |
Description | Data deposited in or computed by PubChem | |
DSSTOX Substance ID |
DTXSID50176204 | |
Record name | 1,2-Diethynylbenzene | |
Source | EPA DSSTox | |
URL | https://comptox.epa.gov/dashboard/DTXSID50176204 | |
Description | DSSTox provides a high quality public chemistry resource for supporting improved predictive toxicology. | |
Molecular Weight |
126.15 g/mol | |
Source | PubChem | |
URL | https://pubchem.ncbi.nlm.nih.gov | |
Description | Data deposited in or computed by PubChem | |
CAS No. |
26776-82-9, 21792-52-9 | |
Record name | Benzene, o-diethynyl-, dimer | |
Source | CAS Common Chemistry | |
URL | https://commonchemistry.cas.org/detail?cas_rn=26776-82-9 | |
Description | CAS Common Chemistry is an open community resource for accessing chemical information. Nearly 500,000 chemical substances from CAS REGISTRY cover areas of community interest, including common and frequently regulated chemicals, and those relevant to high school and undergraduate chemistry classes. This chemical information, curated by our expert scientists, is provided in alignment with our mission as a division of the American Chemical Society. | |
Explanation | The data from CAS Common Chemistry is provided under a CC-BY-NC 4.0 license, unless otherwise stated. | |
Record name | 1,2-Diethynylbenzene | |
Source | ChemIDplus | |
URL | https://pubchem.ncbi.nlm.nih.gov/substance/?source=chemidplus&sourceid=0021792529 | |
Description | ChemIDplus is a free, web search system that provides access to the structure and nomenclature authority files used for the identification of chemical substances cited in National Library of Medicine (NLM) databases, including the TOXNET system. | |
Record name | 1,2-Diethynylbenzene | |
Source | EPA DSSTox | |
URL | https://comptox.epa.gov/dashboard/DTXSID50176204 | |
Description | DSSTox provides a high quality public chemistry resource for supporting improved predictive toxicology. | |
Synthesis routes and methods
Procedure details
Retrosynthesis Analysis
AI-Powered Synthesis Planning: Our tool employs the Template_relevance Pistachio, Template_relevance Bkms_metabolic, Template_relevance Pistachio_ringbreaker, Template_relevance Reaxys, Template_relevance Reaxys_biocatalysis model, leveraging a vast database of chemical reactions to predict feasible synthetic routes.
One-Step Synthesis Focus: Specifically designed for one-step synthesis, it provides concise and direct routes for your target compounds, streamlining the synthesis process.
Accurate Predictions: Utilizing the extensive PISTACHIO, BKMS_METABOLIC, PISTACHIO_RINGBREAKER, REAXYS, REAXYS_BIOCATALYSIS database, our tool offers high-accuracy predictions, reflecting the latest in chemical research and data.
Strategy Settings
Precursor scoring | Relevance Heuristic |
---|---|
Min. plausibility | 0.01 |
Model | Template_relevance |
Template Set | Pistachio/Bkms_metabolic/Pistachio_ringbreaker/Reaxys/Reaxys_biocatalysis |
Top-N result to add to graph | 6 |
Feasible Synthetic Routes
Q1: What is the molecular formula and weight of 1,2-diethynylbenzene?
A1: The molecular formula of this compound is C10H6. Its molecular weight is 126.15 g/mol.
Q2: What spectroscopic techniques are commonly used to characterize this compound?
A2: this compound is commonly characterized by various spectroscopic techniques, including:
- Infrared (IR) Spectroscopy: IR spectroscopy identifies the characteristic stretching frequency of the C≡C bond in alkynes, including this compound. [, , ]
- Nuclear Magnetic Resonance (NMR) Spectroscopy: NMR provides information about the hydrogen and carbon environments within the molecule, aiding in structural confirmation. [, ]
- Mass Spectrometry (MS): MS helps determine the molecular weight and fragmentation pattern of this compound, confirming its identity and purity. []
Q3: How stable is this compound under ambient conditions?
A3: this compound is generally stable under ambient conditions, but it can undergo polymerization or oxidation upon prolonged exposure to air or heat. []
Q4: Can you elaborate on the thermal reactivity of this compound and its derivatives?
A4: this compound derivatives, particularly enediynes, exhibit interesting thermal reactivities. They undergo exothermic Bergman cyclization reactions, forming highly reactive diradicals that can abstract hydrogen atoms from surrounding molecules. [, , ]
Q5: Are there any notable catalytic applications of this compound or its derivatives?
A6: While this compound itself is not a widely used catalyst, its derivatives, especially those incorporating transition metals, have shown promise in various catalytic reactions. For instance, copper(I) complexes with this compound-based ligands have been studied as potential catalysts for the monooxygenation of phenols, mimicking the activity of the enzyme tyrosinase. []
Q6: Has computational chemistry been employed to study this compound and its reactivity?
A7: Yes, computational methods like Density Functional Theory (DFT) and Moller-Plesset second-order perturbation theory (MP2) have been crucial in understanding the reactivity of this compound derivatives. These studies help predict reaction pathways, estimate activation energies for processes like the Bergman cyclization, and elucidate the influence of substituents on reaction rates. [, , ]
Q7: How do substituents on the benzene ring of this compound influence its reactivity?
A8: Substituents significantly affect the reactivity of this compound, particularly in reactions like the Bergman cyclization. For example, electron-withdrawing groups like nitro (-NO2) and aldehyde (-CHO) at the ortho position decrease the activation energy for cyclization, making the reaction more favorable. []
Q8: How do steric effects influence the reactivity of this compound derivatives?
A9: Steric hindrance plays a crucial role in determining the preferred reaction pathway. Bulky substituents can hinder the classic C1-C6 Bergman cyclization, favoring alternative reactions like the C1-C5 cyclization, leading to the formation of different products. []
Q9: How is this compound commonly synthesized?
A9: this compound can be synthesized using Sonogashira coupling reactions, which involve the palladium-catalyzed coupling of 1,2-diiodobenzene or 1,2-dibromobenzene with a terminal alkyne in the presence of a copper co-catalyst and an amine base.
Q10: What are some interesting reactions involving this compound?
A10: this compound is a versatile building block for complex molecules. Some noteworthy reactions include:
- Glaser Coupling: This reaction allows for the dimerization of this compound, creating macrocyclic structures with interesting properties. []
- Hydrophosphination: Adding phosphines to the triple bonds of this compound can generate phosphine ligands useful in coordination chemistry and catalysis. [, , ]
- Frustrated Lewis Pair (FLP) Reactions: FLPs, typically composed of a Lewis acid and a Lewis base, can react with this compound in various ways, leading to the formation of heterocycles or zwitterionic species. []
Q11: Can this compound form complexes with transition metals?
A12: Yes, this compound readily coordinates to various transition metals. The alkyne moieties can act as either σ-donors or π-acceptors, forming stable metal complexes. [, , ]
Q12: What are some interesting examples of metal complexes containing this compound?
A13: this compound forms complexes with a variety of metals, including copper, silver, iron, and manganese. These complexes exhibit diverse structural motifs and electronic properties, making them intriguing for various applications. [, , , ]
Q13: Is there any relevance of this compound in astrochemistry?
A14: this compound and its derivative, ethynylbenzene, have been detected in the Taurus Molecular Cloud (TMC-1), suggesting their potential role in the formation of polycyclic aromatic hydrocarbons (PAHs) in interstellar environments. [, ]
Disclaimer and Information on In-Vitro Research Products
Please be aware that all articles and product information presented on BenchChem are intended solely for informational purposes. The products available for purchase on BenchChem are specifically designed for in-vitro studies, which are conducted outside of living organisms. In-vitro studies, derived from the Latin term "in glass," involve experiments performed in controlled laboratory settings using cells or tissues. It is important to note that these products are not categorized as medicines or drugs, and they have not received approval from the FDA for the prevention, treatment, or cure of any medical condition, ailment, or disease. We must emphasize that any form of bodily introduction of these products into humans or animals is strictly prohibited by law. It is essential to adhere to these guidelines to ensure compliance with legal and ethical standards in research and experimentation.