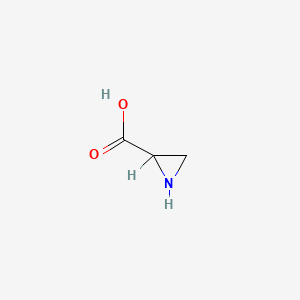
Aziridine-2-carboxylic acid
Overview
Description
Aziridine-2-carboxylic acid is a heterocyclic organic compound characterized by a three-membered aziridine ring and a carboxylic acid functional group.
Mechanism of Action
Target of Action
Aziridine-2-carboxylic acid primarily targets Protein Disulfide Isomerase A1 (PDIA1), a type of enzyme that contains catalytically active thiol groups . PDIA1 usually resides in the endoplasmic reticulum (ER) but can migrate to the cell surface during malignant transformation . The major biological function of intercellular PDIs is to correct the 3D structure of native proteins synthesized in the ER .
Mode of Action
This compound interacts with its target, PDIA1, through a nucleophilic ring-opening reaction . This reaction mainly proceeds with ring opening and generation of alkylated products . The high strain energy of the aziridine ring promotes high reactivity of aziridines toward nucleophiles . Therefore, this compound derivatives activated with an electron-withdrawing group would be more reactive toward the thiol group of the cysteine in comparison to the “classical” thiol-trapping agents .
Biochemical Pathways
This compound affects the biochemical pathways involving PDIA1 . PDIs of the extracellular matrix of cancer cells are antibody targets during immune-mediated tumor destruction . Therefore, the inhibition of PDIA1 by this compound could potentially disrupt these pathways and their downstream effects .
Pharmacokinetics
It’s known that aziridines are inherently strained, making them attractive for study in terms of reactivity and pharmacodynamic action .
Result of Action
The molecular and cellular effects of this compound’s action primarily involve the inhibition of PDIA1 . This inhibition could potentially lead to the disruption of the 3D structure correction of native proteins synthesized in the ER . Moreover, this compound derivatives have demonstrated confirmed pharmacological activity, including antitumor, antimicrobial, and antibacterial effects .
Action Environment
The action, efficacy, and stability of this compound can be influenced by various environmental factors. For instance, nucleophilic ring-opening reactions of this compound with N- and O-nucleophiles take place only in acidic solutions, while reactions with S-nucleophiles occur irrespective of the pH and at a significantly higher reaction rate . Therefore, the physiological pH and the presence of different nucleophiles in the environment can significantly impact the action of this compound .
Biochemical Analysis
Biochemical Properties
Aziridine-2-carboxylic acid derivatives have been synthesized and tested as PDIA1 inhibitors . These derivatives interact with enzymes, proteins, and other biomolecules, particularly the thiol groups of cancer cell surface proteins . The high reactivity of aziridines towards nucleophiles, such as thiol groups, is primarily due to the high strain energy of the aziridine ring .
Cellular Effects
This compound has shown to possess surprisingly low toxicity, which is speculated to be due to its selective alkylation of only thiol groups of cancer cell surface proteins . This selective alkylation can influence cell function, including impacts on cell signaling pathways, gene expression, and cellular metabolism .
Molecular Mechanism
The mechanism of action of this compound involves nucleophilic ring-opening reactions with N- and O-nucleophiles, which occur only in acidic solutions, while reactions with S-nucleophiles occur irrespective of the pH and at a significantly higher reaction rate . This leads to changes in gene expression and enzyme inhibition or activation .
Temporal Effects in Laboratory Settings
It is known that the compound’s stability and degradation, as well as any long-term effects on cellular function, are influenced by its high reactivity towards nucleophiles .
Dosage Effects in Animal Models
It is known that the compound has a surprisingly low toxicity, suggesting that it may have a high threshold effect and limited adverse effects at high doses .
Subcellular Localization
It is known that the compound’s high reactivity towards nucleophiles may influence its targeting signals or post-translational modifications that direct it to specific compartments or organelles .
Preparation Methods
Synthetic Routes and Reaction Conditions: Aziridine-2-carboxylic acid can be synthesized through several methods:
Aziridination: This involves the reaction of imines with carbenes or nitrenes to form the aziridine ring.
Gabriel-Cromwell Cyclization: This method involves the cyclization of amino alcohols.
Diels-Alder Cycloaddition: This reaction involves the cycloaddition of azirines.
Baldwin Rearrangement: This method involves the rearrangement of specific precursors to form the aziridine ring.
Industrial Production Methods: Industrial production of this compound typically involves large-scale aziridination reactions, optimized for yield and purity. The reaction conditions are carefully controlled to ensure the formation of the desired product with minimal by-products .
Chemical Reactions Analysis
Aziridine-2-carboxylic acid undergoes various chemical reactions, including:
Nucleophilic Ring-Opening Reactions: These reactions involve the opening of the aziridine ring by nucleophiles such as thiols, amines, and alcohols.
Oxidation and Reduction Reactions: this compound can be oxidized or reduced under specific conditions to form different products.
Substitution Reactions: The aziridine ring can undergo substitution reactions with various electrophiles, leading to the formation of substituted aziridine derivatives.
Common Reagents and Conditions:
Nucleophiles: Thiols, amines, alcohols.
Oxidizing Agents: Hydrogen peroxide, potassium permanganate.
Reducing Agents: Sodium borohydride, lithium aluminum hydride.
Major Products Formed:
Ring-Opened Products: Amino acids, alkanolamines.
Substituted Aziridines: Various substituted aziridine derivatives.
Scientific Research Applications
Aziridine-2-carboxylic acid has numerous applications in scientific research:
Medicinal Chemistry: It is used as a building block for the synthesis of various biologically active compounds, including anticancer and antimicrobial agents.
Synthetic Organic Chemistry: It serves as an intermediate in the synthesis of complex molecules, including amino acids and peptides.
Biological Research: this compound derivatives are studied for their potential as enzyme inhibitors and immunomodulatory agents.
Industrial Applications: It is used in the production of polymers and coatings, where its unique reactivity is leveraged to enhance material properties.
Comparison with Similar Compounds
Aziridine-2-carboxylic acid can be compared with other aziridine derivatives such as:
Aziridine-2,3-dicarboxylic acid: This compound has an additional carboxylic acid group, which affects its reactivity and applications.
Aziridine-2-carboxamide:
Imexon and Azimexon: These derivatives are also used as anticancer agents and have shown significant biological activity.
Uniqueness: this compound is unique due to its combination of a highly strained aziridine ring and a carboxylic acid functional group. This combination imparts distinct reactivity and makes it a valuable intermediate in the synthesis of various biologically active compounds .
Biological Activity
Aziridine-2-carboxylic acid (Az-COOH) is a compound of significant interest due to its biological activities, particularly as a reactive amino acid unit and potential therapeutic agent. This article summarizes the key findings related to its biological activity, including its mechanisms of action, potential applications in medicinal chemistry, and relevant case studies.
Chemical Structure and Reactivity
This compound features a three-membered aziridine ring, which is highly reactive towards nucleophiles. This unique structure allows it to serve as a valuable scaffold in the design of irreversible inhibitors for cysteine proteinases. The aziridine moiety exhibits reactivity that surpasses that of activated double bonds, making it a potent candidate for selective chemical reactions in biological systems .
Inhibition of Cysteine Proteinases
this compound has been shown to inhibit cysteine proteinases effectively. For instance, it has demonstrated a second-order rate constant of for the inactivation of papain, indicating its strong reactivity towards cysteine thiol groups . This specificity is crucial as it minimizes off-target effects compared to other electrophiles like N-ethylmaleimide.
Protein Disulfide Isomerase (PDI) Inhibition
Recent studies have identified Az-COOH derivatives as potent inhibitors of protein disulfide isomerases (PDIs), specifically PDIA1 and PDIA3. The inhibitory activity was assessed using an insulin reduction assay, revealing effective inhibition at low micromolar concentrations . The binding sites on PDIA1 were characterized using nuclear magnetic resonance (NMR) spectroscopy, highlighting the involvement of specific cysteine residues in the covalent binding process.
Pharmacological Studies
This compound and its derivatives have shown promising pharmacological properties:
- Anti-Cancer Activity : Compounds derived from Az-COOH have exhibited anti-cancer effects through their action on PDIs, which are implicated in cancer cell proliferation and survival.
- Anti-Thrombotic Effects : The inhibition of PDIs also suggests potential applications in thrombotic disorders, as these enzymes play a role in platelet function and coagulation pathways .
Case Study 1: Sulphonamides of this compound
A series of sulphonamides derived from Az-COOH were synthesized and evaluated for their inhibitory effects on PDIs. The results indicated that these compounds could serve as lead candidates for developing new anti-cancer and anti-thrombotic therapies. The study emphasized the importance of structural modifications to enhance biological activity .
Case Study 2: Reactive Amino Acid Applications
Research highlighted the utility of Az-COOH as a reactive amino acid unit in synthesizing irreversible inhibitors. Its ability to selectively alkylate thiol groups without affecting histidine residues presents a unique advantage in drug design .
Summary Table of Biological Activities
Properties
IUPAC Name |
aziridine-2-carboxylic acid | |
---|---|---|
Source | PubChem | |
URL | https://pubchem.ncbi.nlm.nih.gov | |
Description | Data deposited in or computed by PubChem | |
InChI |
InChI=1S/C3H5NO2/c5-3(6)2-1-4-2/h2,4H,1H2,(H,5,6) | |
Source | PubChem | |
URL | https://pubchem.ncbi.nlm.nih.gov | |
Description | Data deposited in or computed by PubChem | |
InChI Key |
WBGBOXYJYPVLQJ-UHFFFAOYSA-N | |
Source | PubChem | |
URL | https://pubchem.ncbi.nlm.nih.gov | |
Description | Data deposited in or computed by PubChem | |
Canonical SMILES |
C1C(N1)C(=O)O | |
Source | PubChem | |
URL | https://pubchem.ncbi.nlm.nih.gov | |
Description | Data deposited in or computed by PubChem | |
Molecular Formula |
C3H5NO2 | |
Source | PubChem | |
URL | https://pubchem.ncbi.nlm.nih.gov | |
Description | Data deposited in or computed by PubChem | |
DSSTOX Substance ID |
DTXSID50968924 | |
Record name | Aziridine-2-carboxylic acid | |
Source | EPA DSSTox | |
URL | https://comptox.epa.gov/dashboard/DTXSID50968924 | |
Description | DSSTox provides a high quality public chemistry resource for supporting improved predictive toxicology. | |
Molecular Weight |
87.08 g/mol | |
Source | PubChem | |
URL | https://pubchem.ncbi.nlm.nih.gov | |
Description | Data deposited in or computed by PubChem | |
CAS No. |
54080-06-7 | |
Record name | Aziridine-2-carboxylic acid | |
Source | ChemIDplus | |
URL | https://pubchem.ncbi.nlm.nih.gov/substance/?source=chemidplus&sourceid=0054080067 | |
Description | ChemIDplus is a free, web search system that provides access to the structure and nomenclature authority files used for the identification of chemical substances cited in National Library of Medicine (NLM) databases, including the TOXNET system. | |
Record name | Aziridine-2-carboxylic acid | |
Source | EPA DSSTox | |
URL | https://comptox.epa.gov/dashboard/DTXSID50968924 | |
Description | DSSTox provides a high quality public chemistry resource for supporting improved predictive toxicology. | |
Retrosynthesis Analysis
AI-Powered Synthesis Planning: Our tool employs the Template_relevance Pistachio, Template_relevance Bkms_metabolic, Template_relevance Pistachio_ringbreaker, Template_relevance Reaxys, Template_relevance Reaxys_biocatalysis model, leveraging a vast database of chemical reactions to predict feasible synthetic routes.
One-Step Synthesis Focus: Specifically designed for one-step synthesis, it provides concise and direct routes for your target compounds, streamlining the synthesis process.
Accurate Predictions: Utilizing the extensive PISTACHIO, BKMS_METABOLIC, PISTACHIO_RINGBREAKER, REAXYS, REAXYS_BIOCATALYSIS database, our tool offers high-accuracy predictions, reflecting the latest in chemical research and data.
Strategy Settings
Precursor scoring | Relevance Heuristic |
---|---|
Min. plausibility | 0.01 |
Model | Template_relevance |
Template Set | Pistachio/Bkms_metabolic/Pistachio_ringbreaker/Reaxys/Reaxys_biocatalysis |
Top-N result to add to graph | 6 |
Feasible Synthetic Routes
Disclaimer and Information on In-Vitro Research Products
Please be aware that all articles and product information presented on BenchChem are intended solely for informational purposes. The products available for purchase on BenchChem are specifically designed for in-vitro studies, which are conducted outside of living organisms. In-vitro studies, derived from the Latin term "in glass," involve experiments performed in controlled laboratory settings using cells or tissues. It is important to note that these products are not categorized as medicines or drugs, and they have not received approval from the FDA for the prevention, treatment, or cure of any medical condition, ailment, or disease. We must emphasize that any form of bodily introduction of these products into humans or animals is strictly prohibited by law. It is essential to adhere to these guidelines to ensure compliance with legal and ethical standards in research and experimentation.