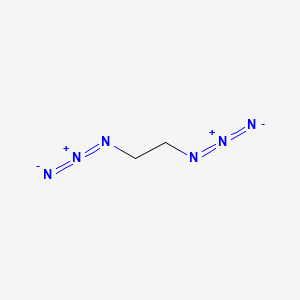
1,2-Diazidoethane
Overview
Description
1,2-Diazidoethane is an organic compound with the molecular formula C2H4N6 It is characterized by the presence of two azido groups (-N3) attached to adjacent carbon atoms in an ethane backbone
Mechanism of Action
Target of Action
1,2-Diazidoethane, also known as Azide-C2-Azide, is primarily used in the synthesis of Antibody-Drug Conjugates (ADCs) . The primary targets of this compound are the antibodies to which the ADC cytotoxin is attached .
Mode of Action
The compound acts as a cleavable ADC linker . It facilitates the attachment of an ADC cytotoxin to an antibody . This interaction results in the formation of ADCs, which are designed to target specific cells, such as cancer cells, in the body .
Biochemical Pathways
It is known that the compound plays a crucial role in the formation of adcs . These ADCs, once formed, can interact with various biochemical pathways depending on the specific antibody and cytotoxin used.
Pharmacokinetics
As a chemical reagent used in the synthesis of adcs, its bioavailability would be determined by the properties of the resulting adcs .
Result of Action
The primary result of this compound’s action is the formation of ADCs . These ADCs are designed to deliver cytotoxins specifically to target cells, thereby reducing the impact on healthy cells . The exact molecular and cellular effects would depend on the specific ADC formed.
Action Environment
The action, efficacy, and stability of this compound can be influenced by various environmental factors. These may include the conditions under which the compound is stored and used, such as temperature and pH . It’s important to note that this compound is a flammable substance and should be kept away from open flames and heat sources .
Biochemical Analysis
Biochemical Properties
1,2-Diazidoethane is typically used in organic synthesis for N-radical reactions, cyclization reactions, and nucleophilic substitution reactions . It also plays a role in the preparation of other compounds, such as fluorescent dyes and high polymer compounds .
Molecular Mechanism
It is known to participate in N-radical reactions, cyclization reactions, and nucleophilic substitution reactions
Temporal Effects in Laboratory Settings
Upon UV irradiation, this compound forms imidazoles, as indicated by NMR spectroscopy and GC-MS analysis This suggests that the effects of this compound can change over time under certain conditions
Preparation Methods
Synthetic Routes and Reaction Conditions
1,2-Diazidoethane can be synthesized through the reaction of 1,2-dibromoethane with sodium azide. The reaction typically involves the following steps:
- Dissolve sodium azide in water.
- Add 1,2-dibromoethane dropwise to the solution at room temperature.
- Heat the mixture to reflux at approximately 80°C for 24 hours.
- Extract the product with ethyl ether and dry it with magnesium sulfate .
Industrial Production Methods
While specific industrial production methods for this compound are not well-documented, the laboratory synthesis method described above can be scaled up for larger production. The key considerations for industrial production would include ensuring safety measures due to the explosive nature of azides and optimizing reaction conditions for higher yields.
Chemical Reactions Analysis
Types of Reactions
1,2-Diazidoethane undergoes various chemical reactions, including:
Thermal Decomposition: Heating this compound can lead to the formation of nitrogen gas and other decomposition products such as hydrogen cyanide and formaldimine.
Photochemical Reactions: UV irradiation of this compound can result in the formation of imidazoles and other nitrogen-containing heterocycles.
Common Reagents and Conditions
Sodium Azide: Used in the synthesis of this compound.
UV Light: Utilized in photochemical reactions to induce the formation of various products.
Major Products Formed
Nitrogen Gas: A common product of thermal decomposition.
Imidazoles: Formed through photochemical reactions in aqueous solutions.
Scientific Research Applications
1,2-Diazidoethane has several applications in scientific research:
Organic Synthesis: It serves as a precursor for the synthesis of nitrogen-containing heterocycles, such as imidazoles.
Materials Science: The compound’s azido groups can be used in the development of new materials with unique properties.
Chemical Research: It is used in studies involving the formation and characterization of diimines and other reactive intermediates.
Comparison with Similar Compounds
Similar Compounds
1,2-Diaminoethane:
1,2-Dibromoethane: Used as a precursor in the synthesis of 1,2-diazidoethane, it contains two bromine atoms attached to the ethane backbone.
Uniqueness
This compound is unique due to its azido groups, which confer distinct reactivity compared to similar compounds like 1,2-diaminoethane. The presence of azido groups allows for the formation of nitrogen gas and reactive intermediates, making it valuable in organic synthesis and materials science .
Properties
IUPAC Name |
1,2-diazidoethane | |
---|---|---|
Source | PubChem | |
URL | https://pubchem.ncbi.nlm.nih.gov | |
Description | Data deposited in or computed by PubChem | |
InChI |
InChI=1S/C2H4N6/c3-7-5-1-2-6-8-4/h1-2H2 | |
Source | PubChem | |
URL | https://pubchem.ncbi.nlm.nih.gov | |
Description | Data deposited in or computed by PubChem | |
InChI Key |
FEJUFSSWIQWAOC-UHFFFAOYSA-N | |
Source | PubChem | |
URL | https://pubchem.ncbi.nlm.nih.gov | |
Description | Data deposited in or computed by PubChem | |
Canonical SMILES |
C(CN=[N+]=[N-])N=[N+]=[N-] | |
Source | PubChem | |
URL | https://pubchem.ncbi.nlm.nih.gov | |
Description | Data deposited in or computed by PubChem | |
Molecular Formula |
C2H4N6 | |
Source | PubChem | |
URL | https://pubchem.ncbi.nlm.nih.gov | |
Description | Data deposited in or computed by PubChem | |
DSSTOX Substance ID |
DTXSID70212072 | |
Record name | Ethane, 1,2-diazido- | |
Source | EPA DSSTox | |
URL | https://comptox.epa.gov/dashboard/DTXSID70212072 | |
Description | DSSTox provides a high quality public chemistry resource for supporting improved predictive toxicology. | |
Molecular Weight |
112.09 g/mol | |
Source | PubChem | |
URL | https://pubchem.ncbi.nlm.nih.gov | |
Description | Data deposited in or computed by PubChem | |
CAS No. |
629-13-0 | |
Record name | 1,2-Diazidoethane | |
Source | CAS Common Chemistry | |
URL | https://commonchemistry.cas.org/detail?cas_rn=629-13-0 | |
Description | CAS Common Chemistry is an open community resource for accessing chemical information. Nearly 500,000 chemical substances from CAS REGISTRY cover areas of community interest, including common and frequently regulated chemicals, and those relevant to high school and undergraduate chemistry classes. This chemical information, curated by our expert scientists, is provided in alignment with our mission as a division of the American Chemical Society. | |
Explanation | The data from CAS Common Chemistry is provided under a CC-BY-NC 4.0 license, unless otherwise stated. | |
Record name | Ethane, 1,2-diazido- | |
Source | ChemIDplus | |
URL | https://pubchem.ncbi.nlm.nih.gov/substance/?source=chemidplus&sourceid=0000629130 | |
Description | ChemIDplus is a free, web search system that provides access to the structure and nomenclature authority files used for the identification of chemical substances cited in National Library of Medicine (NLM) databases, including the TOXNET system. | |
Record name | Ethane, 1,2-diazido- | |
Source | EPA DSSTox | |
URL | https://comptox.epa.gov/dashboard/DTXSID70212072 | |
Description | DSSTox provides a high quality public chemistry resource for supporting improved predictive toxicology. | |
Retrosynthesis Analysis
AI-Powered Synthesis Planning: Our tool employs the Template_relevance Pistachio, Template_relevance Bkms_metabolic, Template_relevance Pistachio_ringbreaker, Template_relevance Reaxys, Template_relevance Reaxys_biocatalysis model, leveraging a vast database of chemical reactions to predict feasible synthetic routes.
One-Step Synthesis Focus: Specifically designed for one-step synthesis, it provides concise and direct routes for your target compounds, streamlining the synthesis process.
Accurate Predictions: Utilizing the extensive PISTACHIO, BKMS_METABOLIC, PISTACHIO_RINGBREAKER, REAXYS, REAXYS_BIOCATALYSIS database, our tool offers high-accuracy predictions, reflecting the latest in chemical research and data.
Strategy Settings
Precursor scoring | Relevance Heuristic |
---|---|
Min. plausibility | 0.01 |
Model | Template_relevance |
Template Set | Pistachio/Bkms_metabolic/Pistachio_ringbreaker/Reaxys/Reaxys_biocatalysis |
Top-N result to add to graph | 6 |
Feasible Synthetic Routes
Q1: What is the significance of 1,2-diiminoethane and how is it related to 1,2-diazidoethane?
A1: 1,2-Diiminoethane is a key intermediate in the synthesis of imidazoles, a class of nitrogen-containing heterocycles with diverse biological activities. this compound serves as a precursor to 1,2-diiminoethane. Upon UV irradiation, this compound undergoes nitrogen extrusion, forming 1,2-diiminoethane. This process has been observed in cryogenic matrices and supported by computational studies. []
Q2: What are the major decomposition products of this compound at high temperatures?
A2: When heated to 600 °C in a matrix isolation experiment, this compound primarily decomposes into hydrogen cyanide and formaldimine. This decomposition pathway was confirmed through deuterium labeling experiments and computational analysis. []
Q3: Can you elaborate on the synthesis and structural characterization of 1,2-bis(5-carboxyethyl-1H-tetrazolyl)ethane?
A3: 1,2-Bis(5-carboxyethyl-1H-tetrazolyl)ethane, a molecule containing two tetrazole rings, can be synthesized through the reaction of this compound with ethyl cyanoformate. This compound has been characterized using various spectroscopic techniques, including IR, mass spectrometry, and NMR spectroscopy. Additionally, its structure has been confirmed by single-crystal X-ray diffraction, revealing its thermal stability and potential as a polymeric precursor. []
Q4: How does the azido gauche effect influence the conformation of this compound and related molecules?
A4: The azido gauche effect, a stereoelectronic phenomenon, significantly impacts the conformational preference of this compound and similar molecules like N-(2-azidoethyl)ethanamide, 2-azidoethanamine, and 2-azidoethanol. This effect favors the gauche conformation, where the azido groups are closer in space. Theoretical studies using MP2/6-311++G(d,p) calculations have revealed that various factors contribute to the gauche preference, including electrostatic interactions, dispersion forces, orbital interactions, and hydrogen bonding. The relative contributions of these factors depend on the specific molecule and its chemical environment. []
Q5: How does the presence of substituents on the aromatic rings affect the synthesis of 1,2-diaryl-1,2-ethanediamines from 1,2-diaryl-1,2-diazidoethanes?
A5: The introduction of certain substituents, particularly methoxy groups, at the ortho or para positions of the aromatic rings in 1,2-diaryl-1,2-diazidoethanes can lead to unexpected elimination reactions during the reduction process aimed at synthesizing the corresponding 1,2-diaryl-1,2-ethanediamines. This results in the formation of undesirable side products. To overcome this challenge, alternative synthetic routes, such as utilizing aziridine derivatives or reducing the corresponding dioximes, have been employed to successfully obtain the desired diamines. []
Disclaimer and Information on In-Vitro Research Products
Please be aware that all articles and product information presented on BenchChem are intended solely for informational purposes. The products available for purchase on BenchChem are specifically designed for in-vitro studies, which are conducted outside of living organisms. In-vitro studies, derived from the Latin term "in glass," involve experiments performed in controlled laboratory settings using cells or tissues. It is important to note that these products are not categorized as medicines or drugs, and they have not received approval from the FDA for the prevention, treatment, or cure of any medical condition, ailment, or disease. We must emphasize that any form of bodily introduction of these products into humans or animals is strictly prohibited by law. It is essential to adhere to these guidelines to ensure compliance with legal and ethical standards in research and experimentation.