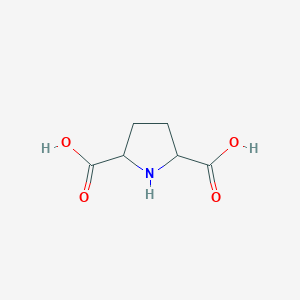
2,5-Pyrrolidinedicarboxylic acid
Overview
Description
2,5-Pyrrolidinedicarboxylic acid is a useful research compound. Its molecular formula is C6H9NO4 and its molecular weight is 159.14 g/mol. The purity is usually 95%.
BenchChem offers high-quality this compound suitable for many research applications. Different packaging options are available to accommodate customers' requirements. Please inquire for more information about this compound including the price, delivery time, and more detailed information at info@benchchem.com.
Mechanism of Action
Target of Action
2,5-Pyrrolidinedicarboxylic acid, also known as 2,5-PDA, is a natural N-heterocyclic compound . It is widely distributed in industrial wastewater and is used as a precursor in the synthesis of pesticides and drugs in agriculture and industries . The primary target of 2,5-PDA is the bacterium Agrobacterium sp. strain YJ-5, which can utilize 2,5-PDA as the sole carbon source for growth .
Mode of Action
The interaction of 2,5-PDA with its target, Agrobacterium sp. strain YJ-5, results in the biodegradation of 2,5-PDA . This bacterium can completely degrade 2,5-PDA within 7 days under optimal growth conditions of temperature (30°C), pH (7.0), and substrate concentration (0.6 mmol −1) .
Biochemical Pathways
The biodegradation of 2,5-PDA by Agrobacterium sp. strain YJ-5 involves the conversion of 2,5-PDA into a new intermediate, 6-hydroxy-2,5-PDA . This conversion occurs when the electron acceptor (2,6-dichlorophenolindophenol) is employed . The identification of this new intermediate provides new insights into the biodegradation pathway of pyridine dicarboxylate .
Pharmacokinetics
strain YJ-5 suggests that this compound can be metabolized and eliminated from the environment
Result of Action
The molecular and cellular effects of 2,5-PDA’s action involve the degradation of the compound into a new intermediate, 6-hydroxy-2,5-PDA . This degradation process is facilitated by the bacterium Agrobacterium sp. strain YJ-5 . The degradation of 2,5-PDA can help in the removal of this compound from industrial wastewater, thereby reducing its environmental impact .
Action Environment
The action of 2,5-PDA is influenced by environmental factors such as temperature, pH, and substrate concentration . The optimal conditions for the growth of Agrobacterium sp. strain YJ-5 and the degradation of 2,5-PDA are a temperature of 30°C, a pH of 7.0, and a substrate concentration of 0.6 mmol −1 . These conditions can influence the efficacy and stability of 2,5-PDA’s action .
Biochemical Analysis
Biochemical Properties
2,5-Pyrrolidinedicarboxylic acid can be utilized as the sole carbon source for growth by certain bacteria, such as the Agrobacterium sp. strain YJ-5 . The compound interacts with various enzymes and proteins within these organisms, facilitating its biodegradation .
Cellular Effects
The effects of this compound on cells are primarily observed in its role as a carbon source. The compound influences cell function by serving as a key nutrient, impacting various cellular processes .
Molecular Mechanism
At the molecular level, this compound is converted into a new intermediate, 6-hydroxy-2,5-Pyrrolidinedicarboxylic acid, by cell extracts of strain YJ-5 . This conversion involves binding interactions with biomolecules and changes in gene expression .
Temporal Effects in Laboratory Settings
In laboratory settings, this compound is completely degraded within 7 days under optimal growth conditions . The compound’s stability, degradation, and long-term effects on cellular function have been observed in in vitro studies .
Metabolic Pathways
This compound is involved in specific metabolic pathways within organisms that can utilize it as a carbon source . It interacts with various enzymes and cofactors during its metabolism .
Biological Activity
2,5-Pyrrolidinedicarboxylic acid (also known as 2,5-PDA) is a compound of significant interest due to its diverse biological activities. This article presents an overview of its biological properties, including antimicrobial, anti-inflammatory, and potential anticancer effects. Additionally, it discusses the mechanisms of action and relevant case studies.
2,5-PDA is a natural N-heterocyclic compound that can be synthesized through various methods, including hydrothermal processes and enzymatic transformations. Its structure allows it to participate in numerous biochemical interactions, making it a valuable candidate for pharmaceutical applications.
Biological Activities
1. Antimicrobial Activity
Recent studies have demonstrated that 2,5-PDA exhibits notable antimicrobial properties. In one study, various derivatives of 2,5-PDA were tested against a range of microorganisms, including Gram-positive and Gram-negative bacteria as well as fungi. The results indicated that certain derivatives showed significant antimicrobial activity, outperforming traditional antibiotics in some cases.
Compound No. | Dose (mg/kg) | % Protection Against Edema | % Inhibition of Plasma PGE2 |
---|---|---|---|
3 | 2.5 | 86.16 ± 0.052 | 59.45 ± 0.050 |
4 | 5.0 | 98.56 ± 0.060 | 80.01 ± 0.058 |
12 | 5.0 | 99.36 ± 0.080 | 86.18 ± 0.071 |
These results suggest that derivatives of 2,5-PDA could serve as effective antimicrobial agents, particularly in the treatment of infections resistant to conventional therapies .
2. Anti-inflammatory Activity
The anti-inflammatory effects of 2,5-PDA have also been investigated extensively. In experimental models of inflammation, compounds derived from 2,5-PDA demonstrated the ability to inhibit the production of pro-inflammatory cytokines and reduce edema significantly.
In a study evaluating the anti-inflammatory potential of various derivatives, it was found that compounds derived from 2,5-PDA could inhibit plasma PGE2 levels effectively, which is a marker for inflammation:
- Most Active Compounds : Compounds such as 6 , 12 , and 10a exhibited the highest levels of anti-inflammatory activity at doses of 5 mg/kg .
3. Anticancer Potential
Emerging research has indicated that 2,5-PDA may possess anticancer properties by modulating cellular pathways involved in tumor growth and metastasis. The compound has been shown to interact with key proteins involved in cancer cell proliferation and survival.
A recent study highlighted its role in blocking the D-DT-induced activation of CD74, a receptor implicated in cancer progression:
- Mechanism : By inhibiting the MIF/CD74 and D-DT/CD74 signaling axes, 2,5-PDA may prevent tumor growth and enhance the efficacy of existing therapies .
Case Studies
- Biodegradation Study : A notable case study focused on the biodegradation of 2,5-PDA by Agrobacterium sp., which utilized the compound as its sole carbon source for growth. The strain was able to completely degrade 2,5-PDA within seven days under optimal conditions (30°C, pH 7). This finding suggests potential applications in bioremediation efforts for environments contaminated with pyridine derivatives .
- Clinical Implications : In clinical settings, the anti-inflammatory properties of derivatives based on 2,5-PDA have shown promise in treating conditions characterized by chronic inflammation, such as arthritis and inflammatory bowel disease.
Scientific Research Applications
Pharmaceutical Applications
Anticancer Activity
Recent studies have highlighted the potential of 2,5-PDCA in cancer therapy. It has been shown to selectively inhibit the activation of CD74 via D-dopachrome tautomerase (D-DT), demonstrating a remarkable 79-fold selectivity over macrophage migration inhibitory factor (MIF) . This selectivity suggests that 2,5-PDCA could be developed into a therapeutic agent targeting specific cancer pathways.
Metal Complexes
The synthesis of metal complexes with 2,5-PDCA has been explored for their antiproliferative properties. For instance, platinum(IV) complexes with acetyl-substituted pyridine ligands exhibit higher in vitro activity against various tumor cell lines compared to traditional chemotherapeutics like cisplatin . These complexes are being investigated for their potential use in targeted cancer therapies.
Biochemical Applications
Biocatalytic Processes
2,5-PDCA can be produced through biocatalytic processes involving the modification of bacteria such as Rhodococcus jostii. This approach utilizes lignin and other renewable feedstocks to produce pyridinedicarboxylic acids efficiently. Such bioconversion methods are significant for sustainable chemical production .
Interaction with Heavy Metals
Research has shown that 2,5-PDCA interacts with various heavy metal ions (e.g., Zn(II), Ni(II), Cu(II)), which can be utilized in environmental chemistry to study metal ion binding and remediation strategies . These interactions are characterized using techniques like polarography and NMR spectroscopy, providing insights into the stability and formation of metal complexes.
Material Science Applications
Synthesis of Polymers
The compound is also explored in the synthesis of biodegradable polymers. Copolymers formed from PDCA derivatives exhibit properties similar to polybutylene adipate co-terephthalate (PBAT), making them suitable replacements for conventional plastics . The flexibility and toughness of these materials hold promise for various applications in packaging and other industries.
Coordination Polymers
2,5-PDCA can be used in the preparation of three-dimensional coordination polymers with lanthanide ions. These materials have potential applications in luminescent devices and as catalysts in organic reactions .
Case Studies
Q & A
Q. Basic: What are the common synthetic routes for preparing 2,5-pyrrolidinedicarboxylic acid, and how do reaction conditions influence product purity?
Answer: The compound is synthesized via hydrolysis of pyrone derivatives in aqueous conditions. Using excess base (e.g., NH4OH) in D2O under reflux yields ammonium pyrrole-2,5-dicarboxylate, with purity dependent on reaction time and temperature. Prolonged heating may cause partial decarboxylation, reducing yields. Optimization involves maintaining pH 8–10 and temperatures below 80°C to minimize side reactions . Alternative methods include catalytic hydrogenation, requiring precise control of catalyst loading (e.g., Pd/C) and hydrogen pressure .
Q. Basic: What analytical techniques are recommended for characterizing this compound and its derivatives?
Answer:
- Structural Confirmation: 1H/13C NMR identifies carboxylate positions and ring puckering. For example, 1H NMR in D2O shows characteristic doublets for α-protons near δ 3.2–3.5 ppm .
- Purity Assessment: HPLC with UV detection (λ = 210 nm) achieves >98% purity, as per industry standards .
- Crystallography: Single-crystal X-ray diffraction resolves stereochemistry in derivatives like N-Boc-protected analogs .
Q. Advanced: How can this compound derivatives be utilized in the design of metal-organic frameworks (MOFs)?
Answer: The dicarboxylate groups act as bridging ligands, coordinating with transition metals (e.g., Pd, Cu) to form porous MOFs. Studies on analogous bipyridine-dicarboxylate systems demonstrate enhanced catalytic activity in cross-coupling reactions when paired with palladium complexes . Computational modeling (DFT) predicts ligand-metal binding energies, guiding MOF design for applications like CO2 capture .
Q. Advanced: What experimental strategies mitigate decarboxylation during synthesis of 2,5-pyrrolidinedicarboxylate salts?
Answer:
- Controlled pH: Maintain pH 8–10 with NH4OH to stabilize carboxylate ions.
- Low-Temperature Reflux: Operate at 60–80°C to reduce thermal degradation.
- Deuterated Solvents: Use D2O for real-time reaction monitoring via NMR .
- Post-Synthesis Treatment: Ion-exchange resins (e.g., Dowex 50WX8) recover free acid without thermal stress .
Q. Methodological: How should researchers address discrepancies in reported solubility data across solvents?
Answer: Standardize solubility studies at 25°C/1 atm using gravimetric or UV-Vis methods. For example, DMSO solubility (1 mg/mL) and aqueous solubility (2 mg/mL) require ultrasonic assistance and warming to 80°C . Discrepancies may arise from polymorphic forms; XRD and TGA confirm crystalline phase consistency .
Q. Data Contradiction: Why do studies report varying yields for derivatives under similar conditions?
Answer: Yield differences stem from stoichiometric variations (e.g., base equivalents) or workup inefficiencies. For instance, incomplete ion-exchange resin treatment leaves ammonium salts, reducing isolated acid yields. Comparative kinetic studies using in situ IR spectroscopy identify rate-limiting steps (e.g., carboxylate protonation) .
Q. Safety & Handling: What precautions are critical for laboratory handling?
Answer:
- PPE: Nitrile gloves and goggles to prevent skin/eye contact (H315/H319 hazards) .
- Storage: Airtight containers at RT, segregated from oxidizers.
- Waste Disposal: Neutralize spills with NaHCO3 before ethanol rinsing; comply with local regulations .
Q. Application-Focused: How are N-protected derivatives applied in peptide mimetics or PROTACs?
Answer: Boc- and Fmoc-protected derivatives serve as rigid proline analogs in peptide backbones, enhancing conformational stability. In PROTACs, the dicarboxylate moiety facilitates linker design for E3 ligase recruitment. Solid-phase synthesis protocols recommend HBTU/HOBt activation for amide bond formation without epimerization .
Q. Comparative Study: How does this compound compare to furan-based analogs in polymer applications?
Answer: The saturated pyrrolidine ring offers greater rotational flexibility than planar furan derivatives, enabling tunable polymer crystallinity. However, furan-based analogs (e.g., 2,5-furandicarboxylic acid) exhibit higher thermal stability (>300°C vs. 250°C), making them preferable for high-temperature applications. DSC/TGA profiles guide selection for biodegradable polyamides .
Q. Computational Modeling: Which methods best predict acid dissociation constants (pKa)?
Answer: DFT calculations (B3LYP/6-311+G(d,p)) with implicit solvation (SMD for water) reproduce experimental pKa values. Multi-conformational analysis accounts for ring puckering effects on protonation energies. Validation against potentiometric titration data (pH 2–12) refines computational parameters .
Properties
IUPAC Name |
pyrrolidine-2,5-dicarboxylic acid | |
---|---|---|
Source | PubChem | |
URL | https://pubchem.ncbi.nlm.nih.gov | |
Description | Data deposited in or computed by PubChem | |
InChI |
InChI=1S/C6H9NO4/c8-5(9)3-1-2-4(7-3)6(10)11/h3-4,7H,1-2H2,(H,8,9)(H,10,11) | |
Source | PubChem | |
URL | https://pubchem.ncbi.nlm.nih.gov | |
Description | Data deposited in or computed by PubChem | |
InChI Key |
ZKXSPYPKBXRBNP-UHFFFAOYSA-N | |
Source | PubChem | |
URL | https://pubchem.ncbi.nlm.nih.gov | |
Description | Data deposited in or computed by PubChem | |
Canonical SMILES |
C1CC(NC1C(=O)O)C(=O)O | |
Source | PubChem | |
URL | https://pubchem.ncbi.nlm.nih.gov | |
Description | Data deposited in or computed by PubChem | |
Molecular Formula |
C6H9NO4 | |
Source | PubChem | |
URL | https://pubchem.ncbi.nlm.nih.gov | |
Description | Data deposited in or computed by PubChem | |
DSSTOX Substance ID |
DTXSID90330328 | |
Record name | 2,5-pyrrolidinedicarboxylic acid | |
Source | EPA DSSTox | |
URL | https://comptox.epa.gov/dashboard/DTXSID90330328 | |
Description | DSSTox provides a high quality public chemistry resource for supporting improved predictive toxicology. | |
Molecular Weight |
159.14 g/mol | |
Source | PubChem | |
URL | https://pubchem.ncbi.nlm.nih.gov | |
Description | Data deposited in or computed by PubChem | |
CAS No. |
72000-65-8 | |
Record name | 2,5-pyrrolidinedicarboxylic acid | |
Source | EPA DSSTox | |
URL | https://comptox.epa.gov/dashboard/DTXSID90330328 | |
Description | DSSTox provides a high quality public chemistry resource for supporting improved predictive toxicology. | |
Retrosynthesis Analysis
AI-Powered Synthesis Planning: Our tool employs the Template_relevance Pistachio, Template_relevance Bkms_metabolic, Template_relevance Pistachio_ringbreaker, Template_relevance Reaxys, Template_relevance Reaxys_biocatalysis model, leveraging a vast database of chemical reactions to predict feasible synthetic routes.
One-Step Synthesis Focus: Specifically designed for one-step synthesis, it provides concise and direct routes for your target compounds, streamlining the synthesis process.
Accurate Predictions: Utilizing the extensive PISTACHIO, BKMS_METABOLIC, PISTACHIO_RINGBREAKER, REAXYS, REAXYS_BIOCATALYSIS database, our tool offers high-accuracy predictions, reflecting the latest in chemical research and data.
Strategy Settings
Precursor scoring | Relevance Heuristic |
---|---|
Min. plausibility | 0.01 |
Model | Template_relevance |
Template Set | Pistachio/Bkms_metabolic/Pistachio_ringbreaker/Reaxys/Reaxys_biocatalysis |
Top-N result to add to graph | 6 |
Feasible Synthetic Routes
Disclaimer and Information on In-Vitro Research Products
Please be aware that all articles and product information presented on BenchChem are intended solely for informational purposes. The products available for purchase on BenchChem are specifically designed for in-vitro studies, which are conducted outside of living organisms. In-vitro studies, derived from the Latin term "in glass," involve experiments performed in controlled laboratory settings using cells or tissues. It is important to note that these products are not categorized as medicines or drugs, and they have not received approval from the FDA for the prevention, treatment, or cure of any medical condition, ailment, or disease. We must emphasize that any form of bodily introduction of these products into humans or animals is strictly prohibited by law. It is essential to adhere to these guidelines to ensure compliance with legal and ethical standards in research and experimentation.