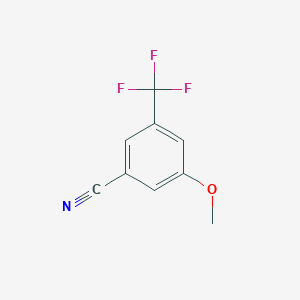
3-Methoxy-5-(trifluoromethyl)benzonitrile
Overview
Description
3-Methoxy-5-(trifluoromethyl)benzonitrile is a chemical compound with the molecular formula C9H6F3NO. Its molecular weight is 201.15 . It’s used in biochemical research .
Molecular Structure Analysis
The InChI code for 3-Methoxy-5-(trifluoromethyl)benzonitrile is 1S/C9H6F3NO/c1-14-8-3-6 (5-13)2-7 (4-8)9 (10,11)12/h2-4H,1H3 .Physical And Chemical Properties Analysis
3-Methoxy-5-(trifluoromethyl)benzonitrile is a solid or semi-solid or liquid at room temperature . Its boiling point is 38°C .Scientific Research Applications
Synthesis of Cholesteryl Ester Transfer Protein (CETP) Inhibitors
This compound is utilized in the synthesis of CETP inhibitors, which are significant in the treatment of hypercholesterolemia. By inhibiting CETP, these compounds raise high-density lipoprotein (HDL) levels and may reduce low-density lipoprotein (LDL) levels, thereby potentially reducing the risk of cardiovascular diseases .
Preparation of Substituted Pyrimidines for Antimicrobial Activity
Researchers use 3-Methoxy-5-(trifluoromethyl)benzonitrile to prepare substituted pyrimidines with dithioacetals. These synthesized compounds exhibit antimicrobial activity, making them valuable in the development of new antibiotics .
Development of Organic Pigments
The compound has been used in the synthesis of new organic pigments. These pigments have been evaluated for their effectiveness as the active medium in solid-state dye lasers, which are used in various technological applications, including medical diagnostics and high-resolution printing .
Safety and Hazards
Mechanism of Action
Target of Action
It’s known that the compound is used in the synthesis of new organic pigments .
Mode of Action
It’s known that the compound undergoes various chemical reactions during the synthesis of new organic pigments
Biochemical Pathways
It’s known that the compound plays a role in the synthesis of new organic pigments , which suggests it may influence pathways related to pigment production or modification.
Pharmacokinetics
The ADME (Absorption, Distribution, Metabolism, and Excretion) properties of 3-Methoxy-5-(trifluoromethyl)benzonitrile are not well-studied. Its bioavailability, metabolism, and excretion patterns remain largely unknown. The compound is a solid at room temperature , which may influence its absorption and distribution characteristics.
Result of Action
Given its role in the synthesis of new organic pigments , it may influence cellular processes related to pigment production or modification.
Action Environment
Environmental factors can significantly influence the action, efficacy, and stability of 3-Methoxy-5-(trifluoromethyl)benzonitrile. For instance, the compound should be stored in a dry, room temperature environment to maintain its stability . Other factors such as pH, presence of other chemicals, and specific conditions of the reaction environment may also affect its action and efficacy.
properties
IUPAC Name |
3-methoxy-5-(trifluoromethyl)benzonitrile | |
---|---|---|
Source | PubChem | |
URL | https://pubchem.ncbi.nlm.nih.gov | |
Description | Data deposited in or computed by PubChem | |
InChI |
InChI=1S/C9H6F3NO/c1-14-8-3-6(5-13)2-7(4-8)9(10,11)12/h2-4H,1H3 | |
Source | PubChem | |
URL | https://pubchem.ncbi.nlm.nih.gov | |
Description | Data deposited in or computed by PubChem | |
InChI Key |
DSMSXBUFGYTQPM-UHFFFAOYSA-N | |
Source | PubChem | |
URL | https://pubchem.ncbi.nlm.nih.gov | |
Description | Data deposited in or computed by PubChem | |
Canonical SMILES |
COC1=CC(=CC(=C1)C(F)(F)F)C#N | |
Source | PubChem | |
URL | https://pubchem.ncbi.nlm.nih.gov | |
Description | Data deposited in or computed by PubChem | |
Molecular Formula |
C9H6F3NO | |
Source | PubChem | |
URL | https://pubchem.ncbi.nlm.nih.gov | |
Description | Data deposited in or computed by PubChem | |
DSSTOX Substance ID |
DTXSID30650532 | |
Record name | 3-Methoxy-5-(trifluoromethyl)benzonitrile | |
Source | EPA DSSTox | |
URL | https://comptox.epa.gov/dashboard/DTXSID30650532 | |
Description | DSSTox provides a high quality public chemistry resource for supporting improved predictive toxicology. | |
Molecular Weight |
201.14 g/mol | |
Source | PubChem | |
URL | https://pubchem.ncbi.nlm.nih.gov | |
Description | Data deposited in or computed by PubChem | |
Product Name |
3-Methoxy-5-(trifluoromethyl)benzonitrile | |
CAS RN |
868167-61-7 | |
Record name | 3-Methoxy-5-(trifluoromethyl)benzonitrile | |
Source | CAS Common Chemistry | |
URL | https://commonchemistry.cas.org/detail?cas_rn=868167-61-7 | |
Description | CAS Common Chemistry is an open community resource for accessing chemical information. Nearly 500,000 chemical substances from CAS REGISTRY cover areas of community interest, including common and frequently regulated chemicals, and those relevant to high school and undergraduate chemistry classes. This chemical information, curated by our expert scientists, is provided in alignment with our mission as a division of the American Chemical Society. | |
Explanation | The data from CAS Common Chemistry is provided under a CC-BY-NC 4.0 license, unless otherwise stated. | |
Record name | 3-Methoxy-5-(trifluoromethyl)benzonitrile | |
Source | EPA DSSTox | |
URL | https://comptox.epa.gov/dashboard/DTXSID30650532 | |
Description | DSSTox provides a high quality public chemistry resource for supporting improved predictive toxicology. | |
Synthesis routes and methods
Procedure details
Retrosynthesis Analysis
AI-Powered Synthesis Planning: Our tool employs the Template_relevance Pistachio, Template_relevance Bkms_metabolic, Template_relevance Pistachio_ringbreaker, Template_relevance Reaxys, Template_relevance Reaxys_biocatalysis model, leveraging a vast database of chemical reactions to predict feasible synthetic routes.
One-Step Synthesis Focus: Specifically designed for one-step synthesis, it provides concise and direct routes for your target compounds, streamlining the synthesis process.
Accurate Predictions: Utilizing the extensive PISTACHIO, BKMS_METABOLIC, PISTACHIO_RINGBREAKER, REAXYS, REAXYS_BIOCATALYSIS database, our tool offers high-accuracy predictions, reflecting the latest in chemical research and data.
Strategy Settings
Precursor scoring | Relevance Heuristic |
---|---|
Min. plausibility | 0.01 |
Model | Template_relevance |
Template Set | Pistachio/Bkms_metabolic/Pistachio_ringbreaker/Reaxys/Reaxys_biocatalysis |
Top-N result to add to graph | 6 |
Feasible Synthetic Routes
Disclaimer and Information on In-Vitro Research Products
Please be aware that all articles and product information presented on BenchChem are intended solely for informational purposes. The products available for purchase on BenchChem are specifically designed for in-vitro studies, which are conducted outside of living organisms. In-vitro studies, derived from the Latin term "in glass," involve experiments performed in controlled laboratory settings using cells or tissues. It is important to note that these products are not categorized as medicines or drugs, and they have not received approval from the FDA for the prevention, treatment, or cure of any medical condition, ailment, or disease. We must emphasize that any form of bodily introduction of these products into humans or animals is strictly prohibited by law. It is essential to adhere to these guidelines to ensure compliance with legal and ethical standards in research and experimentation.