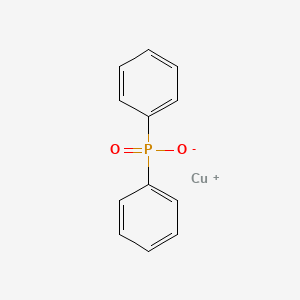
Copper (I) diphenylphosphinate
Overview
Description
Copper(I) diphenylphosphinate (CuDPP, [Ph₂PO₂]⁻Cu⁺) is a copper(I) salt of diphenylphosphinic acid, widely utilized in coordination chemistry and organic synthesis. Its structure comprises a Cu⁺ ion coordinated to the oxygen atoms of the diphenylphosphinate ligand, forming a stable complex with a pseudo-tetrahedral geometry . CuDPP is particularly noted for its role as a co-catalyst in palladium-mediated cross-coupling reactions, where it facilitates desulfitative coupling of thioesters with organostannanes or boronic acids under mild, pH-neutral conditions . This compound exhibits superior catalytic efficiency compared to other copper(I) salts, such as copper(I) thiophenecarboxylate (CuTC), due to its enhanced solubility in polar aprotic solvents like DMF and THF . Applications span the synthesis of α-amino ketones, heterocycles, and fluorinated compounds, with yields often exceeding 70% .
Preparation Methods
Copper-Catalyzed Diphenylation of P(O)-OH Bonds Using Diaryliodonium Salts
A recent advanced synthetic approach involves copper-catalyzed cross-coupling reactions of diphenylphosphinic acid with cyclic diaryliodonium salts to form diphenylphosphinate complexes.
- Catalyst: Copper(I) iodide (CuI) or other copper salts (Cu(OTf)2, CuCl2, Cu powder)
- Substrate: Diphenylphosphinic acid
- Coupling partner: Cyclic diaryliodonium salts
- Base: Triethylamine (Et3N) preferred over inorganic bases for higher yields
- Solvent: Tetrahydrofuran (THF) or toluene
- Temperature: 60–80 °C
- Reaction time: 3–12 hours
Entry | Catalyst | Temperature (°C) | Base | Solvent | Yield (%) |
---|---|---|---|---|---|
1 | Cu(OTf)2 | 80 | K3PO4 | Toluene | 81 |
2 | CuCl2 | 80 | K3PO4 | Toluene | 84 |
3 | Cu powder | 80 | K3PO4 | Toluene | 80 |
4 | Cu(OAc)2 | 80 | K3PO4 | Toluene | Data N/A |
5 | CuI | 80 | K3PO4 | Toluene | 89 |
6 | CuI | 80 | Et3N | THF | 92 |
7 | CuI | 60 | Et3N | THF | 86 |
Data adapted from optimization studies in copper-catalyzed diphenylation of P(O)-OH bonds.
- The copper catalyst facilitates the transfer of diphenylphosphinate groups to the aryl moiety.
- Triethylamine enhances reaction efficiency by avoiding formation of inhibitory inorganic salts.
- The reaction proceeds under mild conditions with good chemoselectivity and broad substrate scope.
Solvothermal Synthesis of Metal Diphenylphosphinate Complexes
A solvothermal method has been reported for the synthesis of metal diphenylphosphinate complexes including copper(I) diphenylphosphinate:
Method Summary:
- Metal salts (e.g., copper salts) and diphenylphosphinic acid are combined in a suitable solvent (often a high-boiling polar solvent).
- The mixture is sealed in an autoclave and heated at elevated temperatures (e.g., 120–180 °C) for several hours to days.
- This method can yield crystalline copper(I) diphenylphosphinate suitable for structural characterization.
- Produces well-defined crystalline materials.
- Enables formation of metal-organic frameworks or coordination polymers.
- Allows control over morphology and particle size.
- Requires specialized equipment (autoclave).
- Longer reaction times.
- Less suitable for large-scale synthesis.
Industrial Scale Preparation Considerations
Industrial production of copper(I) diphenylphosphinate generally follows the direct synthesis route with modifications for scale:
- Use of copper(I) salts with high purity.
- Controlled addition of diphenylphosphinic acid and base under inert atmosphere.
- Optimization of solvent, temperature, and reaction time to maximize yield and purity.
- Purification by recrystallization or filtration to remove impurities.
- Quality control to ensure stability and performance in catalytic or biochemical applications.
Summary Table of Preparation Methods
Method | Copper Source | Ligand Source | Base | Solvent | Conditions | Notes |
---|---|---|---|---|---|---|
Direct synthesis | CuCl (CuI salt) | Diphenylphosphinic acid | Triethylamine | DCM, THF | RT to 80 °C, inert atm. | Most common, simple, requires inert atm. |
Copper-catalyzed diphenylation | CuI, Cu(OTf)2, others | Diphenylphosphinic acid | Et3N | THF, Toluene | 60–80 °C, 3–12 h | High yield, mild conditions, catalytic |
Solvothermal synthesis | Copper salts | Diphenylphosphinic acid | None or base | Polar solvents | 120–180 °C, sealed vessel | Produces crystalline products |
Industrial scale synthesis | CuI, CuCl | Diphenylphosphinic acid | Triethylamine | Organic solvents | Controlled temp, inert atm | Large scale, quality control essential |
Research Findings and Notes
- Triethylamine is preferred over inorganic bases because inorganic salts formed can inhibit complex formation.
- Copper(I) iodide is an effective catalyst for diphenylation reactions, providing yields up to 92% under optimized conditions.
- Solvothermal methods allow for the formation of structurally distinct copper(I) diphenylphosphinate complexes, useful for materials chemistry applications.
- Maintaining copper in the +1 oxidation state is critical; exposure to air or moisture can oxidize copper(I) to copper(II), altering the product.
- Reaction stoichiometry, temperature, and time are key parameters influencing yield and purity.
Scientific Research Applications
Copper (I) diphenylphosphinate has a wide range of applications in scientific research:
Biology: It is studied for its potential biological activities and interactions with biomolecules.
Medicine: Research is ongoing to explore its potential therapeutic applications, including its role in drug development.
Mechanism of Action
The mechanism of action of Copper (I) diphenylphosphinate involves its ability to coordinate with various ligands and participate in redox reactions. It acts as a catalyst by facilitating the transfer of electrons between reactants, thereby lowering the activation energy and increasing the reaction rate. The molecular targets and pathways involved depend on the specific reaction and ligands used .
Comparison with Similar Compounds
Copper(I) Thiophenecarboxylate (CuTC)
CuTC ([C₅H₃SCOO]⁻Cu⁺) shares structural similarities with CuDPP but differs in ligand electronics. While CuDPP’s diphenylphosphinate ligand provides stronger σ-donor and weaker π-acceptor properties, CuTC’s thiophene-carboxylate group offers moderate electron-withdrawing effects. This distinction impacts reactivity: CuDPP outperforms CuTC in cross-coupling reactions, achieving higher yields (e.g., 78% vs. 65% for fluorinated ketones) due to better stabilization of reactive intermediates .
Table 1: Catalytic Performance of CuDPP vs. CuTC
Reaction Type | CuDPP Yield | CuTC Yield | Reference |
---|---|---|---|
Thioester-Stannane Coupling | 78% | 65% | |
Fluorinated Ketone Synthesis | 72% | 58% |
Copper(I) Iodide-Bis(Diphenylphosphino)alkane Complexes
Copper(I) iodide complexes with bis(diphenylphosphino)alkane ligands (e.g., Ph₂P(CH₂)ₙPPh₂) form coordination polymers (CPs) or discrete clusters, contrasting with CuDPP’s monomeric structure. These complexes exhibit tunable photophysical properties, such as thermally activated delayed fluorescence (TADF), driven by ligand-induced metal-metal interactions . For example, [Cu₂I₂(Ph₂PCH₂PPh₂)] shows TADF with a lifetime of 12 μs, whereas CuDPP lacks luminescence due to its non-π-conjugated ligand system .
Table 2: Structural and Functional Differences
Property | CuDPP | CuI-Ph₂P(CH₂)₃PPh₂ Complex |
---|---|---|
Geometry | Monomeric | 1D Coordination Polymer |
Luminescence | None | TADF (λₑₘ = 520 nm) |
Application | Organic Synthesis | Optoelectronics |
Reference |
Silver(I) Diphenylphosphinate
Silver(I) diphenylphosphinate ([Ag(Ph₂PO₂)]ₙ) forms coordination polymers with Ag⁺ centers in 3- or 4-coordinate geometries, unlike CuDPP’s 2-coordinate linear Cu⁺ centers. The Ag complex exhibits catalytic phosphatase-like activity, whereas CuDPP is redox-active in cross-couplings . The softer Lewis acidity of Ag⁺ also reduces its efficacy in desulfitative reactions compared to CuDPP .
Tetrabutylammonium Diphenylphosphinate
This ionic salt ([NBu₄][Ph₂PO₂]) serves as a precursor for phosphinate ligands in click chemistry. Unlike CuDPP, it lacks catalytic activity but enables modular synthesis of triazole-phosphinate hybrids via Cu(I)-catalyzed azide-alkyne cycloaddition (CuAAC). For example, prop-2-ynyl diphenylphosphinate reacts with benzyl azide to form triazole-phosphinates in 88% yield .
Biological Activity
Copper (I) diphenylphosphinate (Cu(I)DP) is an organometallic compound that has garnered attention for its unique biological activities, particularly in the realms of catalysis and potential therapeutic applications. This article explores its biological activity, mechanisms of action, pharmacological profiles, and relevant case studies.
Overview of this compound
Chemical Structure and Properties:
- Molecular Formula: C18H16CuO2P
- Molecular Weight: 335.85 g/mol
- Solubility: Soluble in organic solvents such as dimethylformamide and dichloromethane.
Cu(I)DP is synthesized through the reaction of copper(I) chloride with diphenylphosphinic acid in the presence of a base like triethylamine. This compound serves as a catalyst in various organic reactions, including Suzuki and Sonogashira coupling reactions, highlighting its significance in synthetic chemistry .
Target Interactions
Cu(I)DP interacts with biological targets by binding to lipoylated components of the tricarboxylic acid (TCA) cycle. This interaction disrupts normal cellular metabolism, leading to significant biochemical effects:
- Aggregation of Lipoylated Proteins: Cu(I)DP induces the aggregation of essential proteins involved in energy metabolism.
- Loss of Iron-Sulfur Cluster Proteins: This results in impaired mitochondrial function and increased oxidative stress.
- Induction of Proteotoxic Stress: The compound can trigger cellular stress responses, ultimately leading to cell death .
Pharmacological Profile
Copper is an essential trace element involved in numerous physiological processes. Cu(I)DP exhibits several pharmacological properties:
- Antitumor Activity: Copper complexes, including Cu(I)DP, have shown potential in inhibiting tumor growth through mechanisms involving reactive oxygen species (ROS) generation .
- Antimicrobial Effects: Studies suggest that copper compounds possess inherent antimicrobial properties, making them candidates for infection control .
- Enzyme Mimicry: Cu(I)DP can mimic enzyme activity, enhancing its potential as a therapeutic agent .
Antitumor Activity
A study demonstrated that Cu(I)DP complexes exhibited significant cytotoxicity against melanoma and colon cancer cell lines. The half-inhibitory concentration (IC50) was around 3 μM, indicating potent antitumor effects without notable toxicity during in vivo studies .
Biochemical Applications
Research has highlighted the role of Cu(I)DP in facilitating organic synthesis reactions. For instance, it has been utilized as a catalyst for the diphenylation of P(O)-OH bonds, yielding valuable intermediates for pharmaceuticals and bioactive compounds .
Comparative Analysis
The following table summarizes the biological activity and characteristics of Cu(I)DP compared to other copper compounds:
Compound | Biological Activity | Key Features |
---|---|---|
This compound | Antitumor, antimicrobial | Catalytic efficiency in organic synthesis |
Copper (II) complexes | Antioxidant, enzyme-like activity | Higher systemic toxicity |
Copper (I) chloride | Limited biological activity | Commonly used in catalysis |
Chemical Reactions Analysis
Oxidation and Reduction Reactions
Cu(I)DP participates in redox reactions due to the variable oxidation states of copper. Key pathways include:
Oxidation
-
Reagents : O₂, H₂O₂, or organic peroxides.
-
Products : Copper(II) diphenylphosphinate or higher oxidation state complexes.
-
Mechanism : Oxidation of Cu(I) to Cu(II) occurs via electron transfer, forming reactive intermediates that stabilize through ligand coordination .
Reduction
-
Reagents : NaBH₄, LiAlH₄.
-
Products : Elemental copper (Cu⁰) or Cu(I) complexes with modified ligands.
-
Mechanism : Reduction pathways often involve ligand dissociation, followed by electron transfer to the copper center .
Table 1: Redox Reactions of Cu(I)DP
Reaction Type | Reagents | Conditions | Major Product | Source |
---|---|---|---|---|
Oxidation | H₂O₂ | 80°C, DMF | Cu(II) diphenylphosphinate | |
Reduction | NaBH₄ | RT, THF | Cu⁰ nanoparticles |
Substitution Reactions
The diphenylphosphinate ligand in Cu(I)DP is readily replaced by stronger-field ligands, enabling structural diversification:
-
Common Ligands : Phosphines (e.g., dppf), amines, halides.
-
Conditions : Conducted in dichloromethane or THF under inert atmospheres .
-
Example :
This yields luminescent Cu(I) complexes with thermally activated delayed fluorescence (TADF) .
Table 2: Ligand Substitution Examples
Entering Ligand | Product Structure | Application | Source |
---|---|---|---|
dppf (bis-diphenylphosphinoferrocene) | Cu(I)(dppf) complexes | Catalysis, photophysics | |
I⁻ | CuI aggregates | Coordination polymers |
Coupling Reactions
Cu(I)DP catalyzes cross-coupling reactions, particularly in organic synthesis:
Key Reaction: Diphenylation of P(O)–OH Bonds
-
Substrates : Cyclic diaryliodonium salts + phosphinic acids.
-
Mechanism : Oxidative addition of iodonium salts to Cu(I), followed by ligand exchange and reductive elimination (see Scheme 1) .
Scheme 1: Proposed Catalytic Cycle for Diphenylation
-
Oxidative addition of iodonium salt to Cu(I).
-
Ligand exchange with phosphinic acid.
-
Reductive elimination to form biaryl phosphonate.
Coordination-Driven Aggregation
Cu(I)DP forms polynuclear aggregates depending on stoichiometry and ligand chain length:
-
Stoichiometric Trends :
Table 3: Structural Diversity in Cu(I)DP Aggregates
Stoichiometry (Cu:I) | Aggregate Structure | Ligand Chain Length (m) | Photophysical Property | Source |
---|---|---|---|---|
1:1 | Rhomboid Cu₂I₂ | m = 6 | TADF | |
2:3 | Cubane Cu₄I₄ | m = 8 | N/A |
Stability and Degradation
Q & A
Basic Research Questions
Q. How is copper(I) diphenylphosphinate synthesized, and what characterization methods ensure its purity and structural integrity?
Copper(I) diphenylphosphinate is typically synthesized via ligand exchange or precipitation reactions. For example, in the synthesis of covalent organic frameworks (COFs), it is prepared by reacting copper salts with diphenylphosphinate ligands in solvents like chloroform/acetonitrile mixtures . Characterization involves:
- ¹H/¹³C/³¹P NMR spectroscopy to confirm ligand coordination and purity (e.g., δ ~194.3 ppm for carbonyl groups in thioester reactions) .
- Elemental analysis (e.g., ICP-AES for Cu content, yielding ~3.5% in COF-500-Cu) .
- X-ray crystallography to resolve hydrogen-bonding networks and anion charge distribution (e.g., P—O distances of 1.495–1.503 Å in ammonium diphenylphosphinate structures) .
Q. What are the primary applications of copper(I) diphenylphosphinate in organic synthesis?
It serves as:
- A stoichiometric coupling agent in cross-coupling reactions, such as Liebeskind–Srogl reactions with O-acetylhydroxamic acids, requiring two equivalents for stannane transmetalation .
- A catalyst in click chemistry for synthesizing triazolylmethyl diphenylphosphinate derivatives (63–91% yields under optimized conditions: 60°C, 3 mol% CuSO₄·5H₂O, 5 mol% sodium ascorbate) .
Advanced Research Questions
Q. How do counterion size and reaction conditions influence the structural properties of copper(I) diphenylphosphinate-based materials?
- Counterion effects : Larger anions like diphenylphosphinate (PO₂Ph₂⁻) versus BF₄⁻ reduce framework self-interpenetration in COFs, enhancing elasticity (10× increase in demetalated COFs) .
- Solvent and temperature : Reactions in DMF at 80°C for 24 hours optimize yields (71–78%) in thioester cross-linking for 2-aminoazole synthesis .
Q. What mechanistic insights explain the role of copper(I) diphenylphosphinate in promoting oxidative addition and transmetalation?
- Oxidative addition : Copper(I) facilitates the formation of bridging amido-dimers with O-acetylhydroxamic acids, which are less reactive toward transmetalation, necessitating stoichiometric Cu(I) .
- Ligand stability : Diphenylphosphinate’s bulky aryl groups stabilize copper centers, preventing aggregation and enabling selective catalysis in multi-component reactions .
Q. How can researchers resolve contradictions in reported catalytic efficiencies of copper(I) diphenylphosphinate across different reaction systems?
- Systematic optimization : Vary parameters like solvent polarity (e.g., DMF vs. THF), temperature (80–180°C), and ligand-to-metal ratios .
- Comparative studies : Use kinetic profiling (e.g., HRMS/EI for intermediate tracking) to identify rate-limiting steps in coupling versus cycloaddition reactions .
Q. Methodological Guidance
Q. What frameworks (e.g., FINER, PICOT) are suitable for designing rigorous studies on copper(I) diphenylphosphinate?
- PICOT :
- Population : Reaction systems (e.g., cross-coupling, COF synthesis).
- Intervention : Varying Cu(I) loading, solvent, or counterion.
- Comparison : Catalytic vs. stoichiometric regimes.
- Outcome : Yield, selectivity, material elasticity.
- Time : Reaction duration (e.g., 24–72 hours for COF crystallization) .
Properties
IUPAC Name |
copper(1+);diphenylphosphinate | |
---|---|---|
Source | PubChem | |
URL | https://pubchem.ncbi.nlm.nih.gov | |
Description | Data deposited in or computed by PubChem | |
InChI |
InChI=1S/C12H11O2P.Cu/c13-15(14,11-7-3-1-4-8-11)12-9-5-2-6-10-12;/h1-10H,(H,13,14);/q;+1/p-1 | |
Source | PubChem | |
URL | https://pubchem.ncbi.nlm.nih.gov | |
Description | Data deposited in or computed by PubChem | |
InChI Key |
SVIKVYNAINDOJS-UHFFFAOYSA-M | |
Source | PubChem | |
URL | https://pubchem.ncbi.nlm.nih.gov | |
Description | Data deposited in or computed by PubChem | |
Canonical SMILES |
C1=CC=C(C=C1)P(=O)(C2=CC=CC=C2)[O-].[Cu+] | |
Source | PubChem | |
URL | https://pubchem.ncbi.nlm.nih.gov | |
Description | Data deposited in or computed by PubChem | |
Molecular Formula |
C12H10CuO2P | |
Source | PubChem | |
URL | https://pubchem.ncbi.nlm.nih.gov | |
Description | Data deposited in or computed by PubChem | |
DSSTOX Substance ID |
DTXSID40648501 | |
Record name | Copper(1+) diphenylphosphinate | |
Source | EPA DSSTox | |
URL | https://comptox.epa.gov/dashboard/DTXSID40648501 | |
Description | DSSTox provides a high quality public chemistry resource for supporting improved predictive toxicology. | |
Molecular Weight |
280.73 g/mol | |
Source | PubChem | |
URL | https://pubchem.ncbi.nlm.nih.gov | |
Description | Data deposited in or computed by PubChem | |
CAS No. |
1011257-42-3 | |
Record name | Copper(1+) diphenylphosphinate | |
Source | EPA DSSTox | |
URL | https://comptox.epa.gov/dashboard/DTXSID40648501 | |
Description | DSSTox provides a high quality public chemistry resource for supporting improved predictive toxicology. | |
Retrosynthesis Analysis
AI-Powered Synthesis Planning: Our tool employs the Template_relevance Pistachio, Template_relevance Bkms_metabolic, Template_relevance Pistachio_ringbreaker, Template_relevance Reaxys, Template_relevance Reaxys_biocatalysis model, leveraging a vast database of chemical reactions to predict feasible synthetic routes.
One-Step Synthesis Focus: Specifically designed for one-step synthesis, it provides concise and direct routes for your target compounds, streamlining the synthesis process.
Accurate Predictions: Utilizing the extensive PISTACHIO, BKMS_METABOLIC, PISTACHIO_RINGBREAKER, REAXYS, REAXYS_BIOCATALYSIS database, our tool offers high-accuracy predictions, reflecting the latest in chemical research and data.
Strategy Settings
Precursor scoring | Relevance Heuristic |
---|---|
Min. plausibility | 0.01 |
Model | Template_relevance |
Template Set | Pistachio/Bkms_metabolic/Pistachio_ringbreaker/Reaxys/Reaxys_biocatalysis |
Top-N result to add to graph | 6 |
Feasible Synthetic Routes
Disclaimer and Information on In-Vitro Research Products
Please be aware that all articles and product information presented on BenchChem are intended solely for informational purposes. The products available for purchase on BenchChem are specifically designed for in-vitro studies, which are conducted outside of living organisms. In-vitro studies, derived from the Latin term "in glass," involve experiments performed in controlled laboratory settings using cells or tissues. It is important to note that these products are not categorized as medicines or drugs, and they have not received approval from the FDA for the prevention, treatment, or cure of any medical condition, ailment, or disease. We must emphasize that any form of bodily introduction of these products into humans or animals is strictly prohibited by law. It is essential to adhere to these guidelines to ensure compliance with legal and ethical standards in research and experimentation.