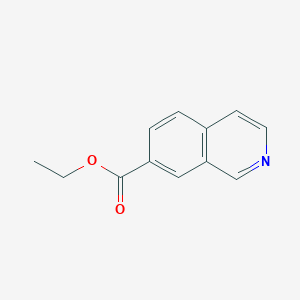
Ethyl isoquinoline-7-carboxylate
Overview
Description
Ethyl isoquinoline-7-carboxylate is a chemical compound belonging to the class of isoquinolines, which are aromatic polycyclic compounds containing an isoquinoline moiety. This moiety consists of a benzene ring fused to a pyridine ring, forming benzo[c]pyridine . Isoquinoline derivatives are known for their diverse applications in medicinal and industrial chemistry .
Mechanism of Action
Target of Action
Ethyl isoquinoline-7-carboxylate is a compound that has been studied for its potential biological activities
Mode of Action
Isoquinoline derivatives have been known to interact with various targets, leading to changes in cellular processes . The specific interactions of this compound with its targets and the resulting changes are areas that warrant further investigation.
Biochemical Pathways
Isoquinoline alkaloids, which include this compound, are synthesized from tyrosine decarboxylated precursor (tyramine or dopamine) which is condensed with a second precursor to form a specific scaffold
Result of Action
Isoquinoline derivatives have been known to exert diverse biological activities
Action Environment
It is known that the synthesis of isoquinoline derivatives can be influenced by various environmental factors
Biochemical Analysis
Biochemical Properties
Ethyl isoquinoline-7-carboxylate plays a significant role in various biochemical reactions. It interacts with several enzymes, proteins, and other biomolecules. For instance, it has been observed to interact with cytochrome P450 enzymes, which are crucial for the metabolism of many drugs. The nature of these interactions often involves the binding of this compound to the active site of the enzyme, potentially inhibiting or modifying its activity .
Cellular Effects
This compound influences various cellular processes. It has been shown to affect cell signaling pathways, gene expression, and cellular metabolism. For example, in cancer cells, this compound can inhibit cell proliferation by interfering with specific signaling pathways such as the MAPK/ERK pathway . Additionally, it can alter gene expression profiles, leading to changes in cellular behavior and function.
Molecular Mechanism
The molecular mechanism of action of this compound involves several key interactions at the molecular level. It binds to specific biomolecules, such as enzymes and receptors, leading to enzyme inhibition or activation. For instance, this compound can inhibit the activity of certain kinases, which are enzymes that play a critical role in cell signaling and regulation . This inhibition can result in altered cellular responses and functions.
Temporal Effects in Laboratory Settings
In laboratory settings, the effects of this compound can change over time. The compound’s stability and degradation are important factors to consider. This compound is relatively stable under standard laboratory conditions, but it may degrade over extended periods or under specific conditions such as high temperatures or exposure to light . Long-term studies have shown that its effects on cellular function can persist, although the extent of these effects may diminish over time.
Dosage Effects in Animal Models
The effects of this compound vary with different dosages in animal models. At lower doses, the compound may exhibit therapeutic effects, such as anti-inflammatory or anticancer properties. At higher doses, it can cause toxic or adverse effects, including hepatotoxicity and nephrotoxicity . It is crucial to determine the appropriate dosage to balance efficacy and safety.
Metabolic Pathways
This compound is involved in several metabolic pathways. It is metabolized primarily in the liver by cytochrome P450 enzymes, leading to the formation of various metabolites . These metabolites can further participate in biochemical reactions, affecting metabolic flux and metabolite levels. The interaction with cofactors such as NADPH is also essential for its metabolism.
Transport and Distribution
Within cells and tissues, this compound is transported and distributed through various mechanisms. It can interact with transporters and binding proteins that facilitate its movement across cellular membranes . The compound’s localization and accumulation in specific tissues can influence its overall activity and effectiveness.
Subcellular Localization
The subcellular localization of this compound is crucial for its activity and function. It can be directed to specific compartments or organelles through targeting signals or post-translational modifications . For example, it may localize to the mitochondria, where it can influence mitochondrial function and energy production.
Preparation Methods
Synthetic Routes and Reaction Conditions
The synthesis of isoquinoline derivatives, including ethyl isoquinoline-7-carboxylate, can be achieved through various methods. One common approach is the Skraup synthesis, which involves the reaction of aniline with glycerol in the presence of an acid medium (such as sulfuric acid) and an oxidizing agent . This method provides good yields of quinoline derivatives.
Other methods include the Friedländer synthesis, which involves the condensation of 2-aminobenzaldehyde with a ketone, and the Pomeranz-Fritsch reaction, which uses benzylamine and glyoxal . These methods can be modified with eco-friendly transition metal-mediated reactions, ultrasound irradiation, or greener protocols .
Industrial Production Methods
Industrial production of isoquinoline derivatives often employs large-scale synthesis methods that prioritize efficiency and yield. These methods may include the use of continuous flow reactors, microwave-assisted synthesis, and solvent-free conditions to minimize environmental impact .
Chemical Reactions Analysis
Types of Reactions
Ethyl isoquinoline-7-carboxylate can undergo various chemical reactions, including:
Oxidation: This reaction involves the addition of oxygen or the removal of hydrogen. Common oxidizing agents include potassium permanganate and chromium trioxide.
Reduction: This reaction involves the addition of hydrogen or the removal of oxygen. Common reducing agents include lithium aluminum hydride and sodium borohydride.
Substitution: This reaction involves the replacement of one functional group with another.
Common Reagents and Conditions
Oxidation: Potassium permanganate in acidic or basic conditions.
Reduction: Lithium aluminum hydride in anhydrous ether.
Substitution: Halogens in the presence of a catalyst or nucleophiles in polar solvents.
Major Products Formed
The major products formed from these reactions depend on the specific conditions and reagents used. For example, oxidation of this compound may yield isoquinoline-7-carboxylic acid, while reduction may produce this compound derivatives with reduced functional groups .
Scientific Research Applications
Ethyl isoquinoline-7-carboxylate has a wide range of applications in scientific research, including:
Comparison with Similar Compounds
Ethyl isoquinoline-7-carboxylate can be compared with other similar compounds, such as:
Quinoline: Another nitrogen-based heterocyclic compound with a similar structure but different biological activities.
Isoquinoline: The parent compound of this compound, with a simpler structure and different reactivity.
Quinoline-4-carboxylic acid: A related compound with a carboxyl group at a different position, leading to different chemical and biological properties.
This compound is unique due to its specific substitution pattern, which influences its reactivity and biological activities .
Properties
IUPAC Name |
ethyl isoquinoline-7-carboxylate | |
---|---|---|
Source | PubChem | |
URL | https://pubchem.ncbi.nlm.nih.gov | |
Description | Data deposited in or computed by PubChem | |
InChI |
InChI=1S/C12H11NO2/c1-2-15-12(14)10-4-3-9-5-6-13-8-11(9)7-10/h3-8H,2H2,1H3 | |
Source | PubChem | |
URL | https://pubchem.ncbi.nlm.nih.gov | |
Description | Data deposited in or computed by PubChem | |
InChI Key |
SLGGUHTWKIMKSM-UHFFFAOYSA-N | |
Source | PubChem | |
URL | https://pubchem.ncbi.nlm.nih.gov | |
Description | Data deposited in or computed by PubChem | |
Canonical SMILES |
CCOC(=O)C1=CC2=C(C=C1)C=CN=C2 | |
Source | PubChem | |
URL | https://pubchem.ncbi.nlm.nih.gov | |
Description | Data deposited in or computed by PubChem | |
Molecular Formula |
C12H11NO2 | |
Source | PubChem | |
URL | https://pubchem.ncbi.nlm.nih.gov | |
Description | Data deposited in or computed by PubChem | |
DSSTOX Substance ID |
DTXSID60627151 | |
Record name | Ethyl isoquinoline-7-carboxylate | |
Source | EPA DSSTox | |
URL | https://comptox.epa.gov/dashboard/DTXSID60627151 | |
Description | DSSTox provides a high quality public chemistry resource for supporting improved predictive toxicology. | |
Molecular Weight |
201.22 g/mol | |
Source | PubChem | |
URL | https://pubchem.ncbi.nlm.nih.gov | |
Description | Data deposited in or computed by PubChem | |
CAS No. |
407623-83-0 | |
Record name | Ethyl isoquinoline-7-carboxylate | |
Source | EPA DSSTox | |
URL | https://comptox.epa.gov/dashboard/DTXSID60627151 | |
Description | DSSTox provides a high quality public chemistry resource for supporting improved predictive toxicology. | |
Retrosynthesis Analysis
AI-Powered Synthesis Planning: Our tool employs the Template_relevance Pistachio, Template_relevance Bkms_metabolic, Template_relevance Pistachio_ringbreaker, Template_relevance Reaxys, Template_relevance Reaxys_biocatalysis model, leveraging a vast database of chemical reactions to predict feasible synthetic routes.
One-Step Synthesis Focus: Specifically designed for one-step synthesis, it provides concise and direct routes for your target compounds, streamlining the synthesis process.
Accurate Predictions: Utilizing the extensive PISTACHIO, BKMS_METABOLIC, PISTACHIO_RINGBREAKER, REAXYS, REAXYS_BIOCATALYSIS database, our tool offers high-accuracy predictions, reflecting the latest in chemical research and data.
Strategy Settings
Precursor scoring | Relevance Heuristic |
---|---|
Min. plausibility | 0.01 |
Model | Template_relevance |
Template Set | Pistachio/Bkms_metabolic/Pistachio_ringbreaker/Reaxys/Reaxys_biocatalysis |
Top-N result to add to graph | 6 |
Feasible Synthetic Routes
Disclaimer and Information on In-Vitro Research Products
Please be aware that all articles and product information presented on BenchChem are intended solely for informational purposes. The products available for purchase on BenchChem are specifically designed for in-vitro studies, which are conducted outside of living organisms. In-vitro studies, derived from the Latin term "in glass," involve experiments performed in controlled laboratory settings using cells or tissues. It is important to note that these products are not categorized as medicines or drugs, and they have not received approval from the FDA for the prevention, treatment, or cure of any medical condition, ailment, or disease. We must emphasize that any form of bodily introduction of these products into humans or animals is strictly prohibited by law. It is essential to adhere to these guidelines to ensure compliance with legal and ethical standards in research and experimentation.