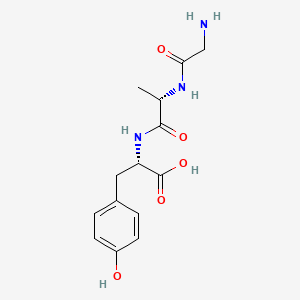
H-Gly-Ala-Tyr-OH
Overview
Description
H-Gly-Ala-Tyr-OH is a tripeptide composed of glycine, alanine, and tyrosine Tripeptides are short chains of amino acids linked by peptide bonds
Mechanism of Action
Target of Action
H-Gly-Ala-Tyr-OH, also known as Gly-Ala-Tyr, is a tripeptide composed of the amino acids glycine, alanine, and tyrosine . The primary targets of this compound are proteins and enzymes that interact with these amino acids. For instance, tyrosine, a proteinogenic amino acid bearing an electron-rich phenol moiety in the side chain, is one of the primary targets for protein oxidation, which plays an essential role in cellular physiology and pathology .
Mode of Action
The interaction of this compound with its targets involves the formation of peptide bonds between the amino acids. This process is facilitated by enzymes such as L-amino acid ligase . The L-amino acid ligase from Bacillus subtilis was selected and coupled with the polyphosphate kinase from Sulfurovum lithotrophicum for regenerating ATP to produce Gly-Ala-Tyr in one pot .
Biochemical Pathways
The synthesis of this compound affects several biochemical pathways. The multi-enzyme coupling reaction system has become a promising biomanufacturing platform for biochemical production . The enzymatic cascade of Gly-Ala-Tyr synthesis was developed by a L-amino acid ligase together with polyphosphate kinase . This process leads to the production of the tripeptide, which can then interact with other molecules and affect downstream biochemical pathways.
Result of Action
The molecular and cellular effects of this compound’s action are primarily related to its role as a building block in protein synthesis. For instance, the dipeptide Tyr-Ala, a functional peptide with typical health benefits, was applied to alleviate oxidative stress in pancreatic islets under hyperglycemic conditions .
Action Environment
The action, efficacy, and stability of this compound can be influenced by various environmental factors. For instance, the pH of the environment can affect the formation of peptide bonds during the synthesis of the compound . Additionally, factors such as temperature and the presence of other molecules can also influence the action of this compound.
Biochemical Analysis
Biochemical Properties
H-Gly-Ala-Tyr-OH participates in a multitude of biochemical reactions. It is synthesized by L-amino acid ligase, an enzyme that condenses two amino acids to form a dipeptide . The L-amino acid ligase from Bacillus subtilis was used in conjunction with polyphosphate kinase from Sulfurovum lithotrophicum for ATP regeneration, leading to the production of this compound .
Cellular Effects
The effects of this compound on cellular processes are diverse. Glycine, one of the constituents of this compound, is critical in the osmoregulation of fishes and shellfishes and improves growth in many farmed animals . There is also an indication that alanine can enhance the growth of aquatic animals .
Molecular Mechanism
The molecular mechanism of this compound involves its interaction with various biomolecules. The L-amino acid ligase from Bacillus subtilis, for instance, interacts with this compound during its synthesis .
Temporal Effects in Laboratory Settings
In laboratory settings, the effects of this compound can change over time. For example, in one study, 40.1 mM of this compound was produced within 3 hours due to efficient ATP regeneration .
Metabolic Pathways
This compound is involved in several metabolic pathways. It is synthesized by L-amino acid ligase, which is part of the peptide synthesis pathway .
Preparation Methods
Synthetic Routes and Reaction Conditions: The synthesis of H-Gly-Ala-Tyr-OH typically involves solid-phase peptide synthesis (SPPS). This method allows for the sequential addition of amino acids to a growing peptide chain anchored to a solid resin. The process involves:
Coupling Reaction: The amino group of glycine is protected with a temporary protecting group such as fluorenylmethyloxycarbonyl (Fmoc). The carboxyl group of glycine is then activated using a coupling reagent like dicyclohexylcarbodiimide (DCC) or benzotriazol-1-yloxytris(dimethylamino)phosphonium hexafluorophosphate (BOP).
Deprotection: The Fmoc group is removed using a base like piperidine, exposing the amino group for the next coupling reaction.
Sequential Addition: Alanine and tyrosine are added sequentially using the same coupling and deprotection steps.
Cleavage: The completed peptide is cleaved from the resin using a strong acid like trifluoroacetic acid (TFA).
Industrial Production Methods: In an industrial setting, the synthesis of this compound can be scaled up using automated peptide synthesizers. These machines automate the repetitive steps of SPPS, allowing for the efficient production of large quantities of peptides with high purity.
Types of Reactions:
Oxidation: this compound can undergo oxidation reactions, particularly at the tyrosine residue, which contains a phenolic hydroxyl group. Common oxidizing agents include hydrogen peroxide and iodine.
Reduction: Reduction reactions are less common for this tripeptide but can occur under specific conditions.
Substitution: The peptide can undergo substitution reactions, particularly at the amino and carboxyl termini.
Common Reagents and Conditions:
Oxidation: Hydrogen peroxide (H₂O₂) in aqueous solution.
Reduction: Sodium borohydride (NaBH₄) in methanol.
Substitution: N-hydroxysuccinimide (NHS) esters in the presence of a base like triethylamine (TEA).
Major Products:
Oxidation: Oxidized tyrosine derivatives.
Reduction: Reduced peptide with modified side chains.
Substitution: Peptide derivatives with substituted functional groups.
Scientific Research Applications
H-Gly-Ala-Tyr-OH has several applications in scientific research:
Biochemistry: Used as a model peptide to study protein folding and interactions.
Pharmacology: Investigated for its potential as a therapeutic agent due to its bioactive properties.
Medicine: Explored for its role in drug delivery systems and as a component of peptide-based vaccines.
Industry: Utilized in the development of peptide-based materials and hydrogels for biomedical applications.
Comparison with Similar Compounds
H-Gly-Ala-Phe-OH: A tripeptide with phenylalanine instead of tyrosine.
H-Gly-Ala-Trp-OH: A tripeptide with tryptophan instead of tyrosine.
H-Gly-Ala-Ser-OH: A tripeptide with serine instead of tyrosine.
Comparison:
H-Gly-Ala-Tyr-OH vs. H-Gly-Ala-Phe-OH: Tyrosine contains a hydroxyl group, making it more polar and capable of forming hydrogen bonds, whereas phenylalanine is more hydrophobic.
This compound vs. H-Gly-Ala-Trp-OH: Tryptophan has a larger indole ring, which can participate in more extensive π-π interactions compared to tyrosine.
This compound vs. H-Gly-Ala-Ser-OH: Serine has a smaller side chain with a hydroxyl group, making it less bulky and more flexible than tyrosine.
This compound stands out due to the unique properties of the tyrosine residue, which can influence the peptide’s interactions and stability.
Properties
IUPAC Name |
2-[2-[(2-aminoacetyl)amino]propanoylamino]-3-(4-hydroxyphenyl)propanoic acid | |
---|---|---|
Details | Computed by LexiChem 2.6.6 (PubChem release 2019.06.18) | |
Source | PubChem | |
URL | https://pubchem.ncbi.nlm.nih.gov | |
Description | Data deposited in or computed by PubChem | |
InChI |
InChI=1S/C14H19N3O5/c1-8(16-12(19)7-15)13(20)17-11(14(21)22)6-9-2-4-10(18)5-3-9/h2-5,8,11,18H,6-7,15H2,1H3,(H,16,19)(H,17,20)(H,21,22) | |
Details | Computed by InChI 1.0.5 (PubChem release 2019.06.18) | |
Source | PubChem | |
URL | https://pubchem.ncbi.nlm.nih.gov | |
Description | Data deposited in or computed by PubChem | |
InChI Key |
QIZJOTQTCAGKPU-UHFFFAOYSA-N | |
Details | Computed by InChI 1.0.5 (PubChem release 2019.06.18) | |
Source | PubChem | |
URL | https://pubchem.ncbi.nlm.nih.gov | |
Description | Data deposited in or computed by PubChem | |
Canonical SMILES |
CC(C(=O)NC(CC1=CC=C(C=C1)O)C(=O)O)NC(=O)CN | |
Details | Computed by OEChem 2.1.5 (PubChem release 2019.06.18) | |
Source | PubChem | |
URL | https://pubchem.ncbi.nlm.nih.gov | |
Description | Data deposited in or computed by PubChem | |
Molecular Formula |
C14H19N3O5 | |
Details | Computed by PubChem 2.1 (PubChem release 2019.06.18) | |
Source | PubChem | |
URL | https://pubchem.ncbi.nlm.nih.gov | |
Description | Data deposited in or computed by PubChem | |
DSSTOX Substance ID |
DTXSID60630367 | |
Record name | Glycylalanyltyrosine | |
Source | EPA DSSTox | |
URL | https://comptox.epa.gov/dashboard/DTXSID60630367 | |
Description | DSSTox provides a high quality public chemistry resource for supporting improved predictive toxicology. | |
Molecular Weight |
309.32 g/mol | |
Details | Computed by PubChem 2.1 (PubChem release 2021.05.07) | |
Source | PubChem | |
URL | https://pubchem.ncbi.nlm.nih.gov | |
Description | Data deposited in or computed by PubChem | |
CAS No. |
92327-84-9 | |
Record name | Glycylalanyltyrosine | |
Source | EPA DSSTox | |
URL | https://comptox.epa.gov/dashboard/DTXSID60630367 | |
Description | DSSTox provides a high quality public chemistry resource for supporting improved predictive toxicology. | |
Retrosynthesis Analysis
AI-Powered Synthesis Planning: Our tool employs the Template_relevance Pistachio, Template_relevance Bkms_metabolic, Template_relevance Pistachio_ringbreaker, Template_relevance Reaxys, Template_relevance Reaxys_biocatalysis model, leveraging a vast database of chemical reactions to predict feasible synthetic routes.
One-Step Synthesis Focus: Specifically designed for one-step synthesis, it provides concise and direct routes for your target compounds, streamlining the synthesis process.
Accurate Predictions: Utilizing the extensive PISTACHIO, BKMS_METABOLIC, PISTACHIO_RINGBREAKER, REAXYS, REAXYS_BIOCATALYSIS database, our tool offers high-accuracy predictions, reflecting the latest in chemical research and data.
Strategy Settings
Precursor scoring | Relevance Heuristic |
---|---|
Min. plausibility | 0.01 |
Model | Template_relevance |
Template Set | Pistachio/Bkms_metabolic/Pistachio_ringbreaker/Reaxys/Reaxys_biocatalysis |
Top-N result to add to graph | 6 |
Feasible Synthetic Routes
Disclaimer and Information on In-Vitro Research Products
Please be aware that all articles and product information presented on BenchChem are intended solely for informational purposes. The products available for purchase on BenchChem are specifically designed for in-vitro studies, which are conducted outside of living organisms. In-vitro studies, derived from the Latin term "in glass," involve experiments performed in controlled laboratory settings using cells or tissues. It is important to note that these products are not categorized as medicines or drugs, and they have not received approval from the FDA for the prevention, treatment, or cure of any medical condition, ailment, or disease. We must emphasize that any form of bodily introduction of these products into humans or animals is strictly prohibited by law. It is essential to adhere to these guidelines to ensure compliance with legal and ethical standards in research and experimentation.