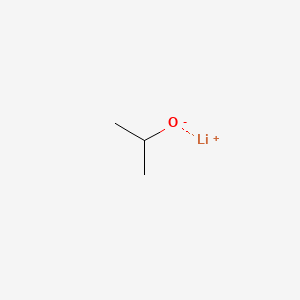
Lithium isopropoxide
Overview
Description
Lithium isopropoxide, also known as lithium 2-propanolate, is an organolithium compound with the chemical formula (CH₃)₂CHOLi. It is a white, hygroscopic solid that is highly reactive and used in various chemical synthesis processes. This compound is known for its strong basicity and nucleophilicity, making it a valuable reagent in organic chemistry.
Mechanism of Action
Target of Action
Lithium isopropoxide, also known as lithium 2-propanolate, is primarily used as a chemical intermediate and for research purposes Lithium compounds, in general, are known to interact with several enzymes that have magnesium as a co-factor .
Mode of Action
Lithium compounds are known to inhibit enzymes that have magnesium as a co-factor . For instance, lithium can inhibit glycogen synthase kinase-3β (GSK-3β), which plays a crucial role in various cellular processes .
Biochemical Pathways
Lithium compounds, including this compound, may affect several biochemical pathways. For instance, lithium is known to influence the dopamine and glutamate pathways, which play a significant role in neurotransmission . Lithium can decrease presynaptic dopamine activity and inactivate postsynaptic G proteins, reducing excitatory neurotransmission in the brain . Furthermore, lithium enhances inhibitory neurotransmission by downregulating the NMDA receptor and inhibiting the myoinositol second messenger system .
Pharmacokinetics
Lithium compounds are generally known to have a narrow therapeutic index, making their pharmacokinetics critical for their therapeutic efficacy .
Result of Action
Lithium compounds are known to have neuroprotective effects . They can reduce cellular damage and enhance resilience in various neurological disorders . Lithium’s neuroprotective properties are thought to be due to its effects on excitotoxic neurotransmission pathways .
Action Environment
The action, efficacy, and stability of this compound can be influenced by various environmental factors. For instance, this compound is classified as a self-heating and skin corrosive substance . Therefore, it needs to be stored in a well-ventilated place and kept cool . It is also important to prevent the product from entering drains .
Preparation Methods
Synthetic Routes and Reaction Conditions: Lithium isopropoxide can be synthesized through the reaction of lithium metal with isopropanol. The reaction is typically carried out under an inert atmosphere to prevent the highly reactive lithium from reacting with moisture or oxygen in the air. The reaction can be represented as follows:
2Li+2(CH3)2CHOH→2(CH3)2CHOLi+H2
The reaction is exothermic and produces hydrogen gas as a byproduct.
Industrial Production Methods: In industrial settings, this compound is often produced by reacting lithium hydroxide with isopropanol. This method is preferred due to the availability and lower reactivity of lithium hydroxide compared to lithium metal. The reaction is as follows:
LiOH+(CH3)2CHOH→(CH3)2CHOLi+H2O
This method also requires careful control of reaction conditions to ensure high purity and yield.
Types of Reactions:
Reduction: this compound is an efficient hydride donor and is used in the reduction of various ketones to alcohols.
Substitution: It can participate in nucleophilic substitution reactions, where it acts as a nucleophile to replace leaving groups in organic molecules.
Deprotonation: Due to its strong basicity, this compound is used to deprotonate weak acids, forming lithium salts and the corresponding alcohols.
Common Reagents and Conditions:
Reagents: Common reagents used with this compound include ketones, esters, and alkyl halides.
Conditions: Reactions involving this compound are typically carried out under anhydrous conditions to prevent hydrolysis and ensure high reactivity.
Major Products:
Reduction of Ketones: Produces secondary alcohols.
Substitution Reactions: Produces substituted organic compounds with this compound as the leaving group.
Scientific Research Applications
Lithium isopropoxide has a wide range of applications in scientific research:
Biology: While not directly used in biological systems, its derivatives and reaction products are often studied for their biological activity.
Medicine: this compound itself is not used in medicine, but its derivatives are explored for potential therapeutic applications.
Comparison with Similar Compounds
- Lithium methoxide
- Lithium ethoxide
- Lithium tert-butoxide
- Potassium tert-butoxide
- Sodium isopropoxide
Comparison:
- Basicity: Lithium isopropoxide is a strong base, similar to lithium tert-butoxide, but less sterically hindered, making it more reactive in certain nucleophilic substitution reactions.
- Reactivity: Compared to lithium methoxide and lithium ethoxide, this compound is more reactive due to the larger size and electron-donating effects of the isopropyl group.
- Applications: While all these compounds are used as bases and nucleophiles, this compound is particularly valued for its balance of reactivity and selectivity in organic synthesis .
This compound is a versatile and valuable compound in both academic and industrial chemistry, with unique properties that make it suitable for a wide range of applications. Its strong basicity and nucleophilicity, combined with its ability to participate in various chemical reactions, make it an essential reagent in the toolkit of chemists.
Properties
IUPAC Name |
lithium;propan-2-olate | |
---|---|---|
Source | PubChem | |
URL | https://pubchem.ncbi.nlm.nih.gov | |
Description | Data deposited in or computed by PubChem | |
InChI |
InChI=1S/C3H7O.Li/c1-3(2)4;/h3H,1-2H3;/q-1;+1 | |
Source | PubChem | |
URL | https://pubchem.ncbi.nlm.nih.gov | |
Description | Data deposited in or computed by PubChem | |
InChI Key |
HAUKUGBTJXWQMF-UHFFFAOYSA-N | |
Source | PubChem | |
URL | https://pubchem.ncbi.nlm.nih.gov | |
Description | Data deposited in or computed by PubChem | |
Canonical SMILES |
[Li+].CC(C)[O-] | |
Source | PubChem | |
URL | https://pubchem.ncbi.nlm.nih.gov | |
Description | Data deposited in or computed by PubChem | |
Molecular Formula |
C3H7LiO | |
Source | PubChem | |
URL | https://pubchem.ncbi.nlm.nih.gov | |
Description | Data deposited in or computed by PubChem | |
DSSTOX Substance ID |
DTXSID40635471 | |
Record name | Lithium propan-2-olate | |
Source | EPA DSSTox | |
URL | https://comptox.epa.gov/dashboard/DTXSID40635471 | |
Description | DSSTox provides a high quality public chemistry resource for supporting improved predictive toxicology. | |
Molecular Weight |
66.1 g/mol | |
Source | PubChem | |
URL | https://pubchem.ncbi.nlm.nih.gov | |
Description | Data deposited in or computed by PubChem | |
CAS No. |
2388-10-5 | |
Record name | Lithium propan-2-olate | |
Source | EPA DSSTox | |
URL | https://comptox.epa.gov/dashboard/DTXSID40635471 | |
Description | DSSTox provides a high quality public chemistry resource for supporting improved predictive toxicology. | |
Record name | Lithium isopropoxide | |
Source | European Chemicals Agency (ECHA) | |
URL | https://echa.europa.eu/information-on-chemicals | |
Description | The European Chemicals Agency (ECHA) is an agency of the European Union which is the driving force among regulatory authorities in implementing the EU's groundbreaking chemicals legislation for the benefit of human health and the environment as well as for innovation and competitiveness. | |
Explanation | Use of the information, documents and data from the ECHA website is subject to the terms and conditions of this Legal Notice, and subject to other binding limitations provided for under applicable law, the information, documents and data made available on the ECHA website may be reproduced, distributed and/or used, totally or in part, for non-commercial purposes provided that ECHA is acknowledged as the source: "Source: European Chemicals Agency, http://echa.europa.eu/". Such acknowledgement must be included in each copy of the material. ECHA permits and encourages organisations and individuals to create links to the ECHA website under the following cumulative conditions: Links can only be made to webpages that provide a link to the Legal Notice page. | |
Retrosynthesis Analysis
AI-Powered Synthesis Planning: Our tool employs the Template_relevance Pistachio, Template_relevance Bkms_metabolic, Template_relevance Pistachio_ringbreaker, Template_relevance Reaxys, Template_relevance Reaxys_biocatalysis model, leveraging a vast database of chemical reactions to predict feasible synthetic routes.
One-Step Synthesis Focus: Specifically designed for one-step synthesis, it provides concise and direct routes for your target compounds, streamlining the synthesis process.
Accurate Predictions: Utilizing the extensive PISTACHIO, BKMS_METABOLIC, PISTACHIO_RINGBREAKER, REAXYS, REAXYS_BIOCATALYSIS database, our tool offers high-accuracy predictions, reflecting the latest in chemical research and data.
Strategy Settings
Precursor scoring | Relevance Heuristic |
---|---|
Min. plausibility | 0.01 |
Model | Template_relevance |
Template Set | Pistachio/Bkms_metabolic/Pistachio_ringbreaker/Reaxys/Reaxys_biocatalysis |
Top-N result to add to graph | 6 |
Feasible Synthetic Routes
Disclaimer and Information on In-Vitro Research Products
Please be aware that all articles and product information presented on BenchChem are intended solely for informational purposes. The products available for purchase on BenchChem are specifically designed for in-vitro studies, which are conducted outside of living organisms. In-vitro studies, derived from the Latin term "in glass," involve experiments performed in controlled laboratory settings using cells or tissues. It is important to note that these products are not categorized as medicines or drugs, and they have not received approval from the FDA for the prevention, treatment, or cure of any medical condition, ailment, or disease. We must emphasize that any form of bodily introduction of these products into humans or animals is strictly prohibited by law. It is essential to adhere to these guidelines to ensure compliance with legal and ethical standards in research and experimentation.