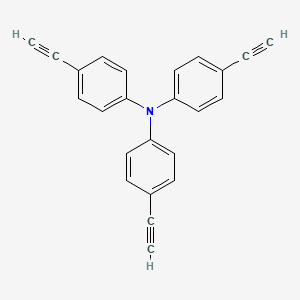
Tris(4-ethynylphenyl)amine
Overview
Description
Tris(4-ethynylphenyl)amine, also known as tris(4-ethynylphenyl)amine, is an aromatic organic compound with three ethynyl groups attached to a phenyl ring. It is used in a variety of applications, including organic synthesis, pharmaceuticals, and materials science. It is also used as an intermediate in the synthesis of other compounds.
Scientific Research Applications
Luminescent Organometallic Networks
Tris(4-ethynylphenyl)amine has been utilized as a ligand in the construction of 3D organometallic networks. One such example involves its use with silver clusters, resulting in a network that exhibits yellowish-green emission at room temperature. This finding suggests potential applications in the field of luminescent materials and organometallic chemistry (Li & Zhang, 2015).
Microporous Polymeric Networks
Research has shown that microporous poly(tri(4-ethynylphenyl)amine) networks can be synthesized with specific surface areas ranging from 500−1100 m²/g. These networks have demonstrated significant control over properties like surface area and pore volume, indicating their usefulness in areas like gas storage and separation (Jiang et al., 2009).
Organoruthenium Dendrimers
Tris(4-ethynylphenyl)amine has been employed as a bridging ligand in the synthesis of novel ruthenium−acetylide dendrimers. These dendrimers exhibit multi-step reversible redox behavior and show a bathochromic shift, indicating their potential in electrochemical applications and molecular electronics (Onitsuka et al., 2008).
Porous Aromatic Frameworks for CO2 Capture
Tris(4-ethynylphenyl)amine-based porous aromatic frameworks have been synthesized for carbon dioxide capture. These frameworks, functionalized with groups like –COOH, –NH2, and –OH, demonstrate enhanced interaction with CO2 molecules, suggesting their effectiveness in CO2 capture and storage technologies (Yuan et al., 2014).
properties
IUPAC Name |
4-ethynyl-N,N-bis(4-ethynylphenyl)aniline | |
---|---|---|
Source | PubChem | |
URL | https://pubchem.ncbi.nlm.nih.gov | |
Description | Data deposited in or computed by PubChem | |
InChI |
InChI=1S/C24H15N/c1-4-19-7-13-22(14-8-19)25(23-15-9-20(5-2)10-16-23)24-17-11-21(6-3)12-18-24/h1-3,7-18H | |
Source | PubChem | |
URL | https://pubchem.ncbi.nlm.nih.gov | |
Description | Data deposited in or computed by PubChem | |
InChI Key |
QGICIDGCKPUALM-UHFFFAOYSA-N | |
Source | PubChem | |
URL | https://pubchem.ncbi.nlm.nih.gov | |
Description | Data deposited in or computed by PubChem | |
Canonical SMILES |
C#CC1=CC=C(C=C1)N(C2=CC=C(C=C2)C#C)C3=CC=C(C=C3)C#C | |
Source | PubChem | |
URL | https://pubchem.ncbi.nlm.nih.gov | |
Description | Data deposited in or computed by PubChem | |
Molecular Formula |
C24H15N | |
Source | PubChem | |
URL | https://pubchem.ncbi.nlm.nih.gov | |
Description | Data deposited in or computed by PubChem | |
DSSTOX Substance ID |
DTXSID70634194 | |
Record name | 4-Ethynyl-N,N-bis(4-ethynylphenyl)aniline | |
Source | EPA DSSTox | |
URL | https://comptox.epa.gov/dashboard/DTXSID70634194 | |
Description | DSSTox provides a high quality public chemistry resource for supporting improved predictive toxicology. | |
Molecular Weight |
317.4 g/mol | |
Source | PubChem | |
URL | https://pubchem.ncbi.nlm.nih.gov | |
Description | Data deposited in or computed by PubChem | |
Product Name |
Tris(4-ethynylphenyl)amine | |
CAS RN |
189178-09-4 | |
Record name | 4-Ethynyl-N,N-bis(4-ethynylphenyl)aniline | |
Source | EPA DSSTox | |
URL | https://comptox.epa.gov/dashboard/DTXSID70634194 | |
Description | DSSTox provides a high quality public chemistry resource for supporting improved predictive toxicology. | |
Record name | Tris(4-ethynylphenyl)amine | |
Source | European Chemicals Agency (ECHA) | |
URL | https://echa.europa.eu/information-on-chemicals | |
Description | The European Chemicals Agency (ECHA) is an agency of the European Union which is the driving force among regulatory authorities in implementing the EU's groundbreaking chemicals legislation for the benefit of human health and the environment as well as for innovation and competitiveness. | |
Explanation | Use of the information, documents and data from the ECHA website is subject to the terms and conditions of this Legal Notice, and subject to other binding limitations provided for under applicable law, the information, documents and data made available on the ECHA website may be reproduced, distributed and/or used, totally or in part, for non-commercial purposes provided that ECHA is acknowledged as the source: "Source: European Chemicals Agency, http://echa.europa.eu/". Such acknowledgement must be included in each copy of the material. ECHA permits and encourages organisations and individuals to create links to the ECHA website under the following cumulative conditions: Links can only be made to webpages that provide a link to the Legal Notice page. | |
Synthesis routes and methods
Procedure details
Retrosynthesis Analysis
AI-Powered Synthesis Planning: Our tool employs the Template_relevance Pistachio, Template_relevance Bkms_metabolic, Template_relevance Pistachio_ringbreaker, Template_relevance Reaxys, Template_relevance Reaxys_biocatalysis model, leveraging a vast database of chemical reactions to predict feasible synthetic routes.
One-Step Synthesis Focus: Specifically designed for one-step synthesis, it provides concise and direct routes for your target compounds, streamlining the synthesis process.
Accurate Predictions: Utilizing the extensive PISTACHIO, BKMS_METABOLIC, PISTACHIO_RINGBREAKER, REAXYS, REAXYS_BIOCATALYSIS database, our tool offers high-accuracy predictions, reflecting the latest in chemical research and data.
Strategy Settings
Precursor scoring | Relevance Heuristic |
---|---|
Min. plausibility | 0.01 |
Model | Template_relevance |
Template Set | Pistachio/Bkms_metabolic/Pistachio_ringbreaker/Reaxys/Reaxys_biocatalysis |
Top-N result to add to graph | 6 |
Feasible Synthetic Routes
Q & A
A: Tris(4-ethynylphenyl)amine serves as a key monomer in the synthesis of microporous poly(arylene ethynylene) frameworks (PAEs). [] These frameworks are generated through the oxidative dimerization of the terminal alkyne groups present in TEPA. The resulting materials exhibit moderate surface areas, ranging from 602 to 715 m2/g, making them potentially useful for applications such as gas storage and separation. []
A: The triphenylamine core in TEPA is vital for enhancing carbon dioxide uptake in porous aromatic frameworks. This core introduces electron-donating basic nitrogen sites within the porous structure, increasing CO2 interaction. [] For instance, a TEPA-based porous aromatic framework demonstrated a CO2 uptake capacity of 4.37 μmol/m2 at 273 K. []
A: Tris(4-ethynylphenyl)amine acts as a bridging ligand in the synthesis of organoruthenium dendrimers. [] The ethynyl groups of TEPA can coordinate to ruthenium centers, facilitating the construction of dendritic structures up to the second generation. These dendrimers display intriguing electrochemical properties. Cyclic voltammetry reveals multistep reversible redox behavior, suggesting electronic communication between the ruthenium centers facilitated by the TEPA bridge. []
A: Tris(4-ethynylphenyl)amine is a key component in the design of luminescent materials. For example, it has been used to create a 3D organometallic network by linking silver clusters (Ag30 and Ag17) via various interactions, including argentophilic interactions and coordination with silver ions. [] This network exhibits yellowish-green emission at room temperature. []
A: Tris(4-ethynylphenyl)amine serves as a building block for hole transport materials in OLEDs. [, ] Researchers have synthesized various TEPA-based materials, including low molecular weight triphenyldiamines, 1,3,5-tris(diarylamino)benzenes, polymeric triphenyldiamines, and triphenylamine networks. [, ] Notably, OLEDs incorporating a polymeric TEPA derivative as the hole transport layer and tris(8-quinolinolato)aluminum as the emitter have achieved a luminance of up to 900 cd/m2 with an onset voltage of 3V. []
A: Tris(4-ethynylphenyl)amine is a crucial monomer in the preparation of hyperbranched polyynes and polytriazoles. [, ] These polymers are synthesized via copper-catalyzed azide-alkyne cycloaddition reactions and Glaser-Hay oxidative coupling conditions, respectively. [, ] The resulting hyperbranched polymers have potential applications in areas like photoluminescence, light refraction, thermal curing, metal complexation, and as precursors for nanostructured magnetoceramics. [, ]
A: The three ethynyl groups in Tris(4-ethynylphenyl)amine make it an ideal candidate for click chemistry, specifically the copper-catalyzed azide-alkyne cycloaddition (CuAAC) reaction. [] This reaction allows for the efficient and modular synthesis of complex macromolecules. For instance, TEPA has been reacted with an azide-tagged copper(I) complex to produce novel hyperbranched NHC-Cu(I) complex-based "click polymers". []
A: Tris(4-ethynylphenyl)amine is employed as an electron donor in conjugated microporous polymers (CMPs) designed for photocatalytic CO2 reduction. [] For example, a CMP combining TEPA with phenanthraquinone as an electron acceptor demonstrated visible-light-driven CO2 reduction to methane (CH4) with high selectivity (>97%). [] The electron-donating nature of TEPA, coupled with the electron-accepting properties of phenanthraquinone, enables efficient charge transfer within the CMP, facilitating the photocatalytic process. []
A: Tris(4-ethynylphenyl)amine serves as a building block for conjugated microporous polymers (CMPs) employed in photocatalytic water splitting for hydrogen evolution. [] The extended conjugation and electron-rich nature of TEPA contribute to the light-harvesting and electron-transfer capabilities of these CMPs, making them promising candidates for sustainable hydrogen production from water. []
A: Tris(4-ethynylphenyl)amine, along with 2,6-diiodo-9,10-anthraquinone, is utilized as a building block in the synthesis of hollow microporous organic networks via Sonogashira coupling using silica spheres as templates. [] These networks, containing both triphenylamine and anthraquinone moieties, demonstrate effective visible light absorption and exhibit catalytic activity in the photochemical oxidative coupling of benzylamines. []
A: Atomistic simulations have been employed to elucidate the micropore structure and surface area of microporous poly(tri(4-ethynylphenyl)amine) networks. [] These simulations, based on experimental characterization data, help in understanding the relationship between the network structure and its gas adsorption properties. []
A: The average monomer strut length in Tris(4-ethynylphenyl)amine-based networks plays a significant role in determining their physical properties. By adjusting this length, fine control over characteristics like BET surface area, Langmuir surface area, micropore surface area, micropore volume, and bulk density can be achieved. []
A: Incorporating functional groups like -COOH, -NH2, and -OH into Tris(4-ethynylphenyl)amine-based porous aromatic frameworks significantly impacts their CO2 capture abilities. [] Notably, the -NH2 functionalized network exhibits the highest isosteric heat of CO2 and CO2/N2 selectivity due to enhanced interactions with CO2 molecules. []
A: Tris(4-ethynylphenyl)amine serves as a key component in the development of fluorescent organic polymers for sensing applications. [] For instance, a triphenylamine-functionalized fluorescent organic polymer has been reported as a "turn-on" fluorescent sensor for Fe3+ ions with high sensitivity and selectivity. []
A: Tris(4-ethynylphenyl)amine is used in the synthesis of conjugated microporous polymers that exhibit aggregation-induced electrochemiluminescence (AIECL). [] For example, TBPE-CMP-1, a CMP incorporating TEPA, shows an impressive ECL efficiency of 1.72%. [] This AIECL property makes these materials promising for applications in sensing, with TBPE-CMP-1 successfully demonstrating the detection of dopamine. []
A: Although specific details are limited, Tris(4-ethynylphenyl)amine plays a role in the synthesis and properties of novel water-soluble terphenylene diethynylene fluorophores. [] The presence of TEPA likely contributes to the fluorophores' solubility and potentially influences their excitation and emission properties.
A: Hyperbranched polyynes derived from Tris(4-ethynylphenyl)amine exhibit excellent thermal stability. [] They are readily curable at temperatures around 150 °C, decompose at temperatures up to ~550 °C, and demonstrate high char yields (up to ~80%) upon pyrolysis at 900 °C. []
A: Complexation of cobalt carbonyls with Tris(4-ethynylphenyl)amine-based hyperbranched polyynes leads to the metallization of these polymers. [] Subsequent ceramization of these metal-polymer complexes produces soft ferromagnetic materials with notable magnetic properties, including high magnetizability (up to ~118 emu/g) and low coercivity (down to ~0.045 kOe). []
A: Trinuclear ruthenium-acetylide complexes incorporating Tris(4-ethynylphenyl)amine as a bridge display stepwise quasi-reversible redox behavior involving three ruthenium-acetylide units and the central triphenylamine unit. [] This behavior contrasts with the two sequential quasi-reversible redox waves observed in their mononuclear counterparts. [] Spectroelectrochemical studies further revealed that the one-electron oxidized triruthenium species is stable and exhibits a distinctive absorption band at 505 nm. [] These findings suggest electronic coupling between the ruthenium centers mediated by the TEPA bridge. []
Disclaimer and Information on In-Vitro Research Products
Please be aware that all articles and product information presented on BenchChem are intended solely for informational purposes. The products available for purchase on BenchChem are specifically designed for in-vitro studies, which are conducted outside of living organisms. In-vitro studies, derived from the Latin term "in glass," involve experiments performed in controlled laboratory settings using cells or tissues. It is important to note that these products are not categorized as medicines or drugs, and they have not received approval from the FDA for the prevention, treatment, or cure of any medical condition, ailment, or disease. We must emphasize that any form of bodily introduction of these products into humans or animals is strictly prohibited by law. It is essential to adhere to these guidelines to ensure compliance with legal and ethical standards in research and experimentation.