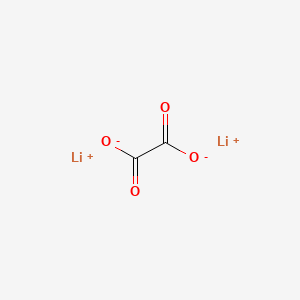
Lithium oxalate
Overview
Description
Lithium Oxalate is an organic compound with the chemical formula Li2C2O4 . It is a salt of lithium metal and oxalic acid . It consists of lithium cations Li+ and oxalate anions C2O2− 4 . It is soluble in water and converts to lithium oxide when heated . It is used as a lithium battery electrolyte and also as an analytical reagent and reducing reagent . Furthermore, it is used as an intermediate in the manufacture of other chemicals such as pharmaceuticals, agrochemicals, dyestuffs, and in organic synthesis .
Synthesis Analysis
One of the methods of synthesis is the reaction of direct neutralization of oxalic acid with lithium hydroxide . The reaction is as follows: 2 LiOH + H2C2O4 → Li2C2O4 + 2 H2O .Molecular Structure Analysis
This compound has a molecular formula of C2Li2O4 . The compound crystallizes in the monoclinic system, with cell parameters a = 3.400 Å, b = 5.156 Å, c = 9.055 Å, β = 95.60°, Z = 4 .Chemical Reactions Analysis
This compound decomposes when heated at 410–500 °C (770–932 °F; 683–773 K): Li2C2O4 → Li2CO3 + CO . All oxalate containing salts generate this compound upon reduction .Physical And Chemical Properties Analysis
This compound is a colorless crystalline solid with a density of 2.12 g/cm^3 . It is soluble in water, with a solubility of 6.6 g per 100 g of water . The molar mass of this compound is 101.90 g·mol^−1 .Scientific Research Applications
1. Enhancing Electrolyte Thermal Stability in Lithium-Ion Batteries
Lithium difluoro(oxalate) borate (LiDFOB) is used to improve the thermal stability of electrolytes in lithium-ion batteries. It aids in forming better solid electrolyte interfaces (SEIs) on both anode and cathode, enhancing cell performance and capacity retention (Xu et al., 2011).
2. Improving Battery Performance
Lithium oxalate acts as a “sacrificial salt” in combination with lithium nickel manganese spinel (LNMO) cathodes, enhancing initial discharge capacity and reducing capacity fade over time in battery cells (Solchenbach et al., 2018).
3. Stabilization of High-Voltage Cathodes
In high-voltage cathode-electrolyte interfaces, lithium bis(oxalato) borate (LiBOB) improves the electrochemical performance of cells, particularly at elevated temperatures (Ha et al., 2013).
4. Electrochemical CO2 Conversion
This compound is identified as a product in the reductive coupling of carbon dioxide using a copper complex. This process holds potential in CO2 utilization strategies, converting it into value-added compounds (Angamuthu et al., 2010).
5. Electrolyte Development for Lithium-Ion Batteries
Research on lithium bis(oxalate)borate-based electrolytes demonstrates the importance of selecting appropriate solvents to optimize the electrochemical performance of these electrolytes in lithium-ion batteries (Cui et al., 2014).
6. Recycling of Lithium-Ion Batteries
This compound is used in novel processes for recycling lithium-ion batteries. One such application involves regenerating Li(Ni1/3Co1/3Mn1/3)O2 cathodes from spent batteries using oxalic acid leaching (Zhang et al., 2018).
7. Improving Electrochemical Performance of Anode Materials
Iron oxalate as an anode material in lithium-ion batteries shows varying performance based on its hydration level.
and crystal structure. Hydrated iron oxalate provides stable force and enlarges interlayer spacing, but its water content can inhibit electrochemical activity, while anhydrate iron oxalate shows better structural stability and performance (Zhang et al., 2021).
8. Recovery of Metals from Spent Batteries
A hydrometallurgical process involving alkali leaching followed by reductive acid leaching using oxalate is effective in recovering cobalt oxalate from spent lithium-ion batteries. This method is environmentally friendly and efficient for valuable metal recovery (Chen et al., 2011).
9. Enhancing Polymer Electrolytes for Batteries
Lithium bis(oxalate)borate (LiBOB) has been used in poly(ethylene oxide)-based composite polymer electrolyte films, showing significant improvements in crystalline structures, thermal properties, and Li+ conductivities. This enhances the potential application of these films as electrolytes in Li-ion polymer batteries (Wu et al., 2011).
10. Investigating Decomposition Mechanisms in Batteries
The decomposition mechanism of lithium bis(oxalate)borate (LiBOB) in the electrolyte of Li–O2 batteries has been studied, revealing that its decomposition leads to this compound formation, impacting the stability and suitability of LiBOB in these battery systems (Lau et al., 2014).
Mechanism of Action
Target of Action
Lithium oxalate is an organic compound with the chemical formula Li2C2O4. It is a salt of lithium metal and oxalic acid . The primary targets of this compound are the lithium cations (Li+) and oxalate anions (C2O2−4) that it consists of .
Mode of Action
The mode of action of this compound involves its interaction with its targets, the lithium cations and oxalate anions. This compound is soluble in water and converts to lithium oxide when heated . This conversion process involves the breaking of bonds between the lithium cations and oxalate anions, leading to changes in their chemical structure .
Biochemical Pathways
It is known that this compound can influence the electron cloud distribution around itself, which can significantly accelerate the release of lithium from the compound . This suggests that this compound may affect biochemical pathways related to electron transfer and energy production.
Safety and Hazards
Future Directions
Lithium Oxalate has potential applications in lithium-ion batteries due to their good reversible capacity, cycling performance, environmental friendliness, and low cost . The distinctive structure of iron oxalate @ nano Ge metal composite provides a fresh pathway to enhance oxalate electrochemical reversible lithium storage activity and develop high-energy electrode material by constructing composite space conductive sites .
properties
IUPAC Name |
dilithium;oxalate | |
---|---|---|
Source | PubChem | |
URL | https://pubchem.ncbi.nlm.nih.gov | |
Description | Data deposited in or computed by PubChem | |
InChI |
InChI=1S/C2H2O4.2Li/c3-1(4)2(5)6;;/h(H,3,4)(H,5,6);;/q;2*+1/p-2 | |
Source | PubChem | |
URL | https://pubchem.ncbi.nlm.nih.gov | |
Description | Data deposited in or computed by PubChem | |
InChI Key |
YNQRWVCLAIUHHI-UHFFFAOYSA-L | |
Source | PubChem | |
URL | https://pubchem.ncbi.nlm.nih.gov | |
Description | Data deposited in or computed by PubChem | |
Canonical SMILES |
[Li+].[Li+].C(=O)(C(=O)[O-])[O-] | |
Source | PubChem | |
URL | https://pubchem.ncbi.nlm.nih.gov | |
Description | Data deposited in or computed by PubChem | |
Molecular Formula |
C2Li2O4 | |
Source | PubChem | |
URL | https://pubchem.ncbi.nlm.nih.gov | |
Description | Data deposited in or computed by PubChem | |
DSSTOX Substance ID |
DTXSID4060288 | |
Record name | Lithium oxalate | |
Source | EPA DSSTox | |
URL | https://comptox.epa.gov/dashboard/DTXSID4060288 | |
Description | DSSTox provides a high quality public chemistry resource for supporting improved predictive toxicology. | |
Molecular Weight |
102.0 g/mol | |
Source | PubChem | |
URL | https://pubchem.ncbi.nlm.nih.gov | |
Description | Data deposited in or computed by PubChem | |
CAS RN |
553-91-3, 30903-87-8 | |
Record name | Lithium oxalate | |
Source | ChemIDplus | |
URL | https://pubchem.ncbi.nlm.nih.gov/substance/?source=chemidplus&sourceid=0000553913 | |
Description | ChemIDplus is a free, web search system that provides access to the structure and nomenclature authority files used for the identification of chemical substances cited in National Library of Medicine (NLM) databases, including the TOXNET system. | |
Record name | Ethanedioic acid, lithium salt (1:2) | |
Source | EPA Chemicals under the TSCA | |
URL | https://www.epa.gov/chemicals-under-tsca | |
Description | EPA Chemicals under the Toxic Substances Control Act (TSCA) collection contains information on chemicals and their regulations under TSCA, including non-confidential content from the TSCA Chemical Substance Inventory and Chemical Data Reporting. | |
Record name | Lithium oxalate | |
Source | EPA DSSTox | |
URL | https://comptox.epa.gov/dashboard/DTXSID4060288 | |
Description | DSSTox provides a high quality public chemistry resource for supporting improved predictive toxicology. | |
Record name | Dilithium oxalate | |
Source | European Chemicals Agency (ECHA) | |
URL | https://echa.europa.eu/substance-information/-/substanceinfo/100.008.232 | |
Description | The European Chemicals Agency (ECHA) is an agency of the European Union which is the driving force among regulatory authorities in implementing the EU's groundbreaking chemicals legislation for the benefit of human health and the environment as well as for innovation and competitiveness. | |
Explanation | Use of the information, documents and data from the ECHA website is subject to the terms and conditions of this Legal Notice, and subject to other binding limitations provided for under applicable law, the information, documents and data made available on the ECHA website may be reproduced, distributed and/or used, totally or in part, for non-commercial purposes provided that ECHA is acknowledged as the source: "Source: European Chemicals Agency, http://echa.europa.eu/". Such acknowledgement must be included in each copy of the material. ECHA permits and encourages organisations and individuals to create links to the ECHA website under the following cumulative conditions: Links can only be made to webpages that provide a link to the Legal Notice page. | |
Record name | Ethanedioic acid, lithium salt (1:?) | |
Source | European Chemicals Agency (ECHA) | |
URL | https://echa.europa.eu/substance-information/-/substanceinfo/100.107.577 | |
Description | The European Chemicals Agency (ECHA) is an agency of the European Union which is the driving force among regulatory authorities in implementing the EU's groundbreaking chemicals legislation for the benefit of human health and the environment as well as for innovation and competitiveness. | |
Explanation | Use of the information, documents and data from the ECHA website is subject to the terms and conditions of this Legal Notice, and subject to other binding limitations provided for under applicable law, the information, documents and data made available on the ECHA website may be reproduced, distributed and/or used, totally or in part, for non-commercial purposes provided that ECHA is acknowledged as the source: "Source: European Chemicals Agency, http://echa.europa.eu/". Such acknowledgement must be included in each copy of the material. ECHA permits and encourages organisations and individuals to create links to the ECHA website under the following cumulative conditions: Links can only be made to webpages that provide a link to the Legal Notice page. | |
Record name | LITHIUM OXALATE | |
Source | FDA Global Substance Registration System (GSRS) | |
URL | https://gsrs.ncats.nih.gov/ginas/app/beta/substances/K737OT0E73 | |
Description | The FDA Global Substance Registration System (GSRS) enables the efficient and accurate exchange of information on what substances are in regulated products. Instead of relying on names, which vary across regulatory domains, countries, and regions, the GSRS knowledge base makes it possible for substances to be defined by standardized, scientific descriptions. | |
Explanation | Unless otherwise noted, the contents of the FDA website (www.fda.gov), both text and graphics, are not copyrighted. They are in the public domain and may be republished, reprinted and otherwise used freely by anyone without the need to obtain permission from FDA. Credit to the U.S. Food and Drug Administration as the source is appreciated but not required. | |
Retrosynthesis Analysis
AI-Powered Synthesis Planning: Our tool employs the Template_relevance Pistachio, Template_relevance Bkms_metabolic, Template_relevance Pistachio_ringbreaker, Template_relevance Reaxys, Template_relevance Reaxys_biocatalysis model, leveraging a vast database of chemical reactions to predict feasible synthetic routes.
One-Step Synthesis Focus: Specifically designed for one-step synthesis, it provides concise and direct routes for your target compounds, streamlining the synthesis process.
Accurate Predictions: Utilizing the extensive PISTACHIO, BKMS_METABOLIC, PISTACHIO_RINGBREAKER, REAXYS, REAXYS_BIOCATALYSIS database, our tool offers high-accuracy predictions, reflecting the latest in chemical research and data.
Strategy Settings
Precursor scoring | Relevance Heuristic |
---|---|
Min. plausibility | 0.01 |
Model | Template_relevance |
Template Set | Pistachio/Bkms_metabolic/Pistachio_ringbreaker/Reaxys/Reaxys_biocatalysis |
Top-N result to add to graph | 6 |
Feasible Synthetic Routes
Q & A
Q1: What is the molecular formula and weight of lithium oxalate?
A1: this compound has the molecular formula Li₂C₂O₄ and a molecular weight of 101.9 g/mol.
Q2: What spectroscopic techniques have been used to characterize this compound?
A2: Researchers have employed various spectroscopic methods to analyze this compound, including Fourier transform infrared spectroscopy (FTIR) [, , ], Raman spectroscopy [, ], X-ray photoelectron spectroscopy (XPS) [, , , , ], nuclear magnetic resonance (NMR) spectroscopy [, , , ], and electron spin resonance (ESR) spectroscopy []. These techniques provide insights into the compound's structure, bonding, and interactions within battery systems.
Q3: How does this compound contribute to the lifespan of anode-free lithium metal batteries (AFLMBs)?
A3: In AFLMBs, this compound acts as a "sacrificial salt" when added to the cathode. Upon oxidation, it decomposes into carbon dioxide (CO₂) and lithium ions [, ]. This process provides a supplementary lithium source, mitigating the loss of active lithium due to dendrite formation and dead lithium buildup, thus improving cycle life [].
Q4: What is the thermal stability of this compound in lithium-ion battery electrolytes?
A4: Research indicates that this compound exhibits poor thermal stability in the presence of lithium hexafluorophosphate (LiPF₆), a common electrolyte salt. When heated in dimethyl carbonate (DMC), a common electrolyte solvent, this compound and LiPF₆ react to produce carbon dioxide, lithium fluoride, and lithium difluorophosphate (LiPO₂F₂) []. This decomposition contributes to the instability of the solid electrolyte interphase (SEI) layer in batteries.
Q5: Does this compound dissolve in lithium-ion battery electrolytes?
A5: this compound exhibits limited solubility in dimethyl carbonate (DMC) compared to other lithium salts found in SEI films. Its solubility is lower than that of lithium methyl carbonate, lithium ethyl carbonate, lithium hydroxide, and lithium fluoride []. This limited solubility influences its behavior and distribution within the battery system.
Q6: How does this compound impact the formation of the solid electrolyte interphase (SEI)?
A6: this compound contributes to SEI formation through its decomposition products. It forms during the initial charging cycles as a result of electrolyte reduction on the graphite anode surface [, ]. The presence of this compound, along with other reduction products like lithium ethylene dicarbonate (LEDC) and lithium fluoride (LiF), influences the SEI's composition and properties, impacting battery performance.
Q7: What is the role of this compound in electrocatalytic carbon dioxide conversion?
A7: Research has demonstrated the use of a copper complex to convert carbon dioxide into oxalate []. In this process, this compound precipitates out of the solution when treated with a soluble lithium salt. This highlights a potential application of this compound in carbon dioxide capture and utilization technologies.
Q8: Are there other applications of this compound besides batteries?
A8: Yes, this compound has found applications in other areas. For instance, it is used as a retention modifier in ion chromatography for separating ammonium ions from other cations in environmental water samples []. Additionally, it has shown potential in the synthesis of lithium niobate (LiNbO₃) ultrafine powders, valuable materials for various electronic applications [].
Q9: How can this compound be recovered from lithium-ion battery waste?
A9: One method for recovering this compound from spent lithium-ion batteries involves leaching with oxalic acid []. This process selectively extracts lithium in the form of this compound, allowing for its separation and potential reuse. This approach contributes to sustainable battery recycling and reduces the environmental impact associated with battery waste.
Disclaimer and Information on In-Vitro Research Products
Please be aware that all articles and product information presented on BenchChem are intended solely for informational purposes. The products available for purchase on BenchChem are specifically designed for in-vitro studies, which are conducted outside of living organisms. In-vitro studies, derived from the Latin term "in glass," involve experiments performed in controlled laboratory settings using cells or tissues. It is important to note that these products are not categorized as medicines or drugs, and they have not received approval from the FDA for the prevention, treatment, or cure of any medical condition, ailment, or disease. We must emphasize that any form of bodily introduction of these products into humans or animals is strictly prohibited by law. It is essential to adhere to these guidelines to ensure compliance with legal and ethical standards in research and experimentation.