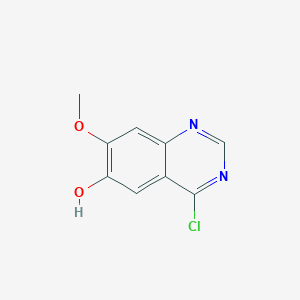
4-Chloro-7-methoxyquinazolin-6-ol
Overview
Description
4-Chloro-7-methoxyquinazolin-6-ol is a heterocyclic aromatic compound with the molecular formula C₉H₇ClN₂O₂. It is a derivative of quinazoline, featuring a chlorine atom at the 4th position, a methoxy group at the 7th position, and a hydroxyl group at the 6th position.
Preparation Methods
Synthetic Routes and Reaction Conditions
4-Chloro-7-methoxyquinazolin-6-ol can be synthesized through several synthetic routes. One common method involves the cyclization of 2-amino-4-chloro-5-methoxybenzoic acid with formamide under acidic conditions. The reaction typically proceeds as follows:
Starting Material: 2-amino-4-chloro-5-methoxybenzoic acid.
Reagent: Formamide.
Conditions: Acidic medium, elevated temperature.
The reaction yields this compound as the final product after purification .
Industrial Production Methods
Industrial production of this compound often involves large-scale synthesis using similar cyclization reactions. The process is optimized for higher yields and purity, with stringent control over reaction conditions such as temperature, pressure, and pH. The use of continuous flow reactors and automated systems ensures consistent quality and efficiency .
Chemical Reactions Analysis
Types of Reactions
4-Chloro-7-methoxyquinazolin-6-ol undergoes various chemical reactions, including:
Substitution Reactions: The chlorine atom at the 4th position can be substituted with other nucleophiles, such as amines or thiols, under appropriate conditions.
Oxidation Reactions: The methoxy group can be oxidized to form corresponding quinazolinone derivatives.
Reduction Reactions: The compound can undergo reduction reactions to modify the quinazoline ring structure.
Common Reagents and Conditions
Substitution: Nucleophiles (e.g., amines, thiols), base (e.g., sodium hydroxide), solvent (e.g., ethanol), elevated temperature.
Oxidation: Oxidizing agents (e.g., potassium permanganate), solvent (e.g., acetone), room temperature.
Reduction: Reducing agents (e.g., lithium aluminum hydride), solvent (e.g., tetrahydrofuran), low temperature.
Major Products
Substitution: Various substituted quinazoline derivatives.
Oxidation: Quinazolinone derivatives.
Reduction: Reduced quinazoline compounds.
Scientific Research Applications
4-Chloro-7-methoxyquinazolin-6-ol has several scientific research applications:
Medicinal Chemistry: It is used as a building block for the synthesis of potential therapeutic agents, including kinase inhibitors and anti-cancer drugs.
Material Science: The compound is explored for its potential use in organic electronics and as a precursor for functional materials.
Biological Studies: It serves as a probe in biochemical assays to study enzyme activities and protein interactions.
Industrial Applications: The compound is used in the synthesis of dyes, pigments, and other specialty chemicals.
Mechanism of Action
The mechanism of action of 4-Chloro-7-methoxyquinazolin-6-ol involves its interaction with specific molecular targets, such as enzymes and receptors. The compound can inhibit the activity of certain kinases by binding to their active sites, thereby blocking signal transduction pathways involved in cell proliferation and survival. This makes it a potential candidate for the development of anti-cancer therapies .
Comparison with Similar Compounds
Similar Compounds
4-Chloro-6-methoxyquinazoline: Similar structure but lacks the hydroxyl group at the 6th position.
7-Methoxyquinazolin-4-ol: Similar structure but lacks the chlorine atom at the 4th position.
4-Chloro-7-methoxyquinoline: Similar structure but has a different ring system (quinoline instead of quinazoline).
Uniqueness
4-Chloro-7-methoxyquinazolin-6-ol is unique due to the presence of both a chlorine atom and a methoxy group on the quinazoline ring, along with a hydroxyl group. This combination of functional groups imparts distinct chemical reactivity and biological activity, making it a versatile compound for various applications .
Properties
IUPAC Name |
4-chloro-7-methoxyquinazolin-6-ol | |
---|---|---|
Source | PubChem | |
URL | https://pubchem.ncbi.nlm.nih.gov | |
Description | Data deposited in or computed by PubChem | |
InChI |
InChI=1S/C9H7ClN2O2/c1-14-8-3-6-5(2-7(8)13)9(10)12-4-11-6/h2-4,13H,1H3 | |
Source | PubChem | |
URL | https://pubchem.ncbi.nlm.nih.gov | |
Description | Data deposited in or computed by PubChem | |
InChI Key |
ZBOFUZBKDHGCAF-UHFFFAOYSA-N | |
Source | PubChem | |
URL | https://pubchem.ncbi.nlm.nih.gov | |
Description | Data deposited in or computed by PubChem | |
Canonical SMILES |
COC1=C(C=C2C(=C1)N=CN=C2Cl)O | |
Source | PubChem | |
URL | https://pubchem.ncbi.nlm.nih.gov | |
Description | Data deposited in or computed by PubChem | |
Molecular Formula |
C9H7ClN2O2 | |
Source | PubChem | |
URL | https://pubchem.ncbi.nlm.nih.gov | |
Description | Data deposited in or computed by PubChem | |
DSSTOX Substance ID |
DTXSID40630880 | |
Record name | 4-Chloro-7-methoxyquinazolin-6-ol | |
Source | EPA DSSTox | |
URL | https://comptox.epa.gov/dashboard/DTXSID40630880 | |
Description | DSSTox provides a high quality public chemistry resource for supporting improved predictive toxicology. | |
Molecular Weight |
210.62 g/mol | |
Source | PubChem | |
URL | https://pubchem.ncbi.nlm.nih.gov | |
Description | Data deposited in or computed by PubChem | |
CAS No. |
574745-97-4 | |
Record name | 4-Chloro-7-methoxyquinazolin-6-ol | |
Source | EPA DSSTox | |
URL | https://comptox.epa.gov/dashboard/DTXSID40630880 | |
Description | DSSTox provides a high quality public chemistry resource for supporting improved predictive toxicology. | |
Synthesis routes and methods
Procedure details
Retrosynthesis Analysis
AI-Powered Synthesis Planning: Our tool employs the Template_relevance Pistachio, Template_relevance Bkms_metabolic, Template_relevance Pistachio_ringbreaker, Template_relevance Reaxys, Template_relevance Reaxys_biocatalysis model, leveraging a vast database of chemical reactions to predict feasible synthetic routes.
One-Step Synthesis Focus: Specifically designed for one-step synthesis, it provides concise and direct routes for your target compounds, streamlining the synthesis process.
Accurate Predictions: Utilizing the extensive PISTACHIO, BKMS_METABOLIC, PISTACHIO_RINGBREAKER, REAXYS, REAXYS_BIOCATALYSIS database, our tool offers high-accuracy predictions, reflecting the latest in chemical research and data.
Strategy Settings
Precursor scoring | Relevance Heuristic |
---|---|
Min. plausibility | 0.01 |
Model | Template_relevance |
Template Set | Pistachio/Bkms_metabolic/Pistachio_ringbreaker/Reaxys/Reaxys_biocatalysis |
Top-N result to add to graph | 6 |
Feasible Synthetic Routes
Disclaimer and Information on In-Vitro Research Products
Please be aware that all articles and product information presented on BenchChem are intended solely for informational purposes. The products available for purchase on BenchChem are specifically designed for in-vitro studies, which are conducted outside of living organisms. In-vitro studies, derived from the Latin term "in glass," involve experiments performed in controlled laboratory settings using cells or tissues. It is important to note that these products are not categorized as medicines or drugs, and they have not received approval from the FDA for the prevention, treatment, or cure of any medical condition, ailment, or disease. We must emphasize that any form of bodily introduction of these products into humans or animals is strictly prohibited by law. It is essential to adhere to these guidelines to ensure compliance with legal and ethical standards in research and experimentation.