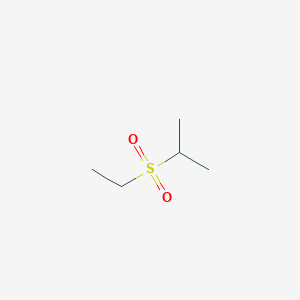
Ethyl Isopropyl Sulfone
Overview
Description
Ethyl Isopropyl Sulfone, also known as 2-(Ethylsulfonyl)propane, is an organosulfur compound with the molecular formula C5H12O2S. It is characterized by the presence of a sulfonyl group (SO2) attached to an ethyl and an isopropyl group. This compound is known for its stability and versatility in various chemical reactions .
Mechanism of Action
Target of Action
Ethyl Isopropyl Sulfone is an organic compound . It is primarily used as a solvent, a catalyst for alcohol condensation reactions, and an intermediate in organic synthesis . Therefore, the primary targets of this compound are the reactants in these chemical reactions.
Mode of Action
As a solvent, this compound dissolves other substances, thereby facilitating chemical reactions . As a catalyst, it speeds up the rate of chemical reactions without being consumed in the process . As an intermediate, it participates in the reaction to form the final product .
Biochemical Pathways
It is known that sulfones, in general, are versatile synthetic intermediates in organic chemistry . They can function as activating, electron-withdrawing substituents in Michael acceptors or as good leaving groups producing a sulfinate anion .
Pharmacokinetics
It is known that this compound is soluble in many organic solvents, such as alcohols, ethers, and ketones, but insoluble in water . This suggests that its bioavailability could be influenced by its solubility properties.
Result of Action
The molecular and cellular effects of this compound’s action are largely dependent on the specific chemical reactions it is involved in. For instance, in the synthesis of polymers, this compound can be used to produce polyethersulfone materials .
Biochemical Analysis
Biochemical Properties
Ethyl Isopropyl Sulfone plays a significant role in biochemical reactions. It interacts with various enzymes, proteins, and other biomolecules. For instance, it can act as a solvent in organic synthesis and as a catalyst in alcohol condensation reactions . The interactions between this compound and these biomolecules are primarily based on its ability to dissolve in organic solvents and its reactivity with other chemical groups.
Cellular Effects
This compound affects various types of cells and cellular processes. It influences cell function by impacting cell signaling pathways, gene expression, and cellular metabolism. For example, it has been observed to affect the stability of supercapacitors at high voltages and temperatures, which suggests its potential impact on cellular electrochemical stability
Molecular Mechanism
The molecular mechanism of this compound involves its interactions at the molecular level, including binding interactions with biomolecules, enzyme inhibition or activation, and changes in gene expression. This compound can act as an electron-withdrawing group, which facilitates various chemical reactions . Its ability to stabilize adjacent carbanions and function as a good leaving group makes it a versatile intermediate in organic synthesis .
Temporal Effects in Laboratory Settings
In laboratory settings, the effects of this compound change over time. The compound is known for its stability and resistance to degradation under standard laboratory conditions . Its long-term effects on cellular function, observed in both in vitro and in vivo studies, indicate that it can form a passivation layer on electrode surfaces, preventing further degradation . This property is particularly useful in maintaining the stability of supercapacitors over extended periods.
Dosage Effects in Animal Models
The effects of this compound vary with different dosages in animal models. At low doses, it has been observed to have minimal toxic effects. At higher doses, it can cause adverse effects, including toxicity and disruption of normal cellular functions . The threshold effects observed in these studies highlight the importance of careful dosage regulation when using this compound in experimental settings.
Metabolic Pathways
This compound is involved in various metabolic pathways. It interacts with enzymes and cofactors that facilitate its breakdown and utilization within the body . The compound’s metabolic pathways include its conversion into other sulfone derivatives, which can then participate in further biochemical reactions. Its effects on metabolic flux and metabolite levels are significant, as it can influence the overall metabolic balance within cells.
Transport and Distribution
Within cells and tissues, this compound is transported and distributed through interactions with transporters and binding proteins . Its localization and accumulation are influenced by its solubility in organic solvents and its reactivity with other chemical groups. The compound’s distribution within cells is crucial for its biochemical activity and overall function.
Subcellular Localization
This compound’s subcellular localization is determined by its targeting signals and post-translational modifications . It is primarily localized in specific compartments or organelles where it can exert its biochemical effects. The compound’s activity and function are influenced by its precise localization within the cell, which is essential for its role in various biochemical processes.
Preparation Methods
Synthetic Routes and Reaction Conditions: Ethyl Isopropyl Sulfone can be synthesized through several methods. One common approach involves the oxidation of thioethers. For instance, the oxidation of ethyl isopropyl sulfide using hydrogen peroxide or other oxidizing agents can yield this compound . Another method involves the sulfonylation of isopropyl ethyl sulfide using sulfonyl chlorides in the presence of a base .
Industrial Production Methods: In industrial settings, the production of this compound often involves the use of continuous flow reactors to ensure consistent quality and yield. The process typically includes the controlled oxidation of thioethers under specific temperature and pressure conditions to maximize efficiency and minimize by-products .
Chemical Reactions Analysis
Types of Reactions: Ethyl Isopropyl Sulfone undergoes various chemical reactions, including:
Oxidation: It can be further oxidized to form sulfoxides and sulfones.
Reduction: It can be reduced back to thioethers under specific conditions.
Substitution: It can participate in nucleophilic substitution reactions where the sulfonyl group acts as a leaving group.
Common Reagents and Conditions:
Oxidation: Hydrogen peroxide, potassium permanganate, or other strong oxidizing agents.
Reduction: Lithium aluminum hydride or other reducing agents.
Substitution: Alkyl halides and bases such as sodium hydroxide.
Major Products Formed:
Oxidation: Sulfoxides and higher sulfones.
Reduction: Thioethers.
Substitution: Various substituted sulfones depending on the nucleophile used.
Scientific Research Applications
Ethyl Isopropyl Sulfone has a wide range of applications in scientific research:
Chemistry: It is used as an intermediate in the synthesis of more complex organosulfur compounds.
Biology: It serves as a model compound for studying the behavior of sulfones in biological systems.
Medicine: It is investigated for its potential use in drug development, particularly in the synthesis of sulfone-based pharmaceuticals.
Industry: It is used in the production of high-performance materials, including polymers and resins
Comparison with Similar Compounds
Dimethyl Sulfone: Similar in structure but with two methyl groups instead of ethyl and isopropyl groups.
Ethyl Methyl Sulfone: Contains an ethyl and a methyl group attached to the sulfonyl group.
Isopropyl Methyl Sulfone: Contains an isopropyl and a methyl group attached to the sulfonyl group.
Uniqueness: Ethyl Isopropyl Sulfone is unique due to its specific combination of ethyl and isopropyl groups, which imparts distinct chemical properties and reactivity compared to other sulfones. This makes it particularly useful in specialized applications where these properties are advantageous .
Properties
IUPAC Name |
2-ethylsulfonylpropane | |
---|---|---|
Source | PubChem | |
URL | https://pubchem.ncbi.nlm.nih.gov | |
Description | Data deposited in or computed by PubChem | |
InChI |
InChI=1S/C5H12O2S/c1-4-8(6,7)5(2)3/h5H,4H2,1-3H3 | |
Source | PubChem | |
URL | https://pubchem.ncbi.nlm.nih.gov | |
Description | Data deposited in or computed by PubChem | |
InChI Key |
RDKKQZIFDSEMNU-UHFFFAOYSA-N | |
Source | PubChem | |
URL | https://pubchem.ncbi.nlm.nih.gov | |
Description | Data deposited in or computed by PubChem | |
Canonical SMILES |
CCS(=O)(=O)C(C)C | |
Source | PubChem | |
URL | https://pubchem.ncbi.nlm.nih.gov | |
Description | Data deposited in or computed by PubChem | |
Molecular Formula |
C5H12O2S | |
Source | PubChem | |
URL | https://pubchem.ncbi.nlm.nih.gov | |
Description | Data deposited in or computed by PubChem | |
DSSTOX Substance ID |
DTXSID50617324 | |
Record name | 2-(Ethanesulfonyl)propane | |
Source | EPA DSSTox | |
URL | https://comptox.epa.gov/dashboard/DTXSID50617324 | |
Description | DSSTox provides a high quality public chemistry resource for supporting improved predictive toxicology. | |
Molecular Weight |
136.21 g/mol | |
Source | PubChem | |
URL | https://pubchem.ncbi.nlm.nih.gov | |
Description | Data deposited in or computed by PubChem | |
CAS No. |
4853-75-2 | |
Record name | 2-(Ethylsulfonyl)propane | |
Source | CAS Common Chemistry | |
URL | https://commonchemistry.cas.org/detail?cas_rn=4853-75-2 | |
Description | CAS Common Chemistry is an open community resource for accessing chemical information. Nearly 500,000 chemical substances from CAS REGISTRY cover areas of community interest, including common and frequently regulated chemicals, and those relevant to high school and undergraduate chemistry classes. This chemical information, curated by our expert scientists, is provided in alignment with our mission as a division of the American Chemical Society. | |
Explanation | The data from CAS Common Chemistry is provided under a CC-BY-NC 4.0 license, unless otherwise stated. | |
Record name | 2-(Ethanesulfonyl)propane | |
Source | EPA DSSTox | |
URL | https://comptox.epa.gov/dashboard/DTXSID50617324 | |
Description | DSSTox provides a high quality public chemistry resource for supporting improved predictive toxicology. | |
Record name | Ethyl Isopropyl Sulfone | |
Source | European Chemicals Agency (ECHA) | |
URL | https://echa.europa.eu/information-on-chemicals | |
Description | The European Chemicals Agency (ECHA) is an agency of the European Union which is the driving force among regulatory authorities in implementing the EU's groundbreaking chemicals legislation for the benefit of human health and the environment as well as for innovation and competitiveness. | |
Explanation | Use of the information, documents and data from the ECHA website is subject to the terms and conditions of this Legal Notice, and subject to other binding limitations provided for under applicable law, the information, documents and data made available on the ECHA website may be reproduced, distributed and/or used, totally or in part, for non-commercial purposes provided that ECHA is acknowledged as the source: "Source: European Chemicals Agency, http://echa.europa.eu/". Such acknowledgement must be included in each copy of the material. ECHA permits and encourages organisations and individuals to create links to the ECHA website under the following cumulative conditions: Links can only be made to webpages that provide a link to the Legal Notice page. | |
Retrosynthesis Analysis
AI-Powered Synthesis Planning: Our tool employs the Template_relevance Pistachio, Template_relevance Bkms_metabolic, Template_relevance Pistachio_ringbreaker, Template_relevance Reaxys, Template_relevance Reaxys_biocatalysis model, leveraging a vast database of chemical reactions to predict feasible synthetic routes.
One-Step Synthesis Focus: Specifically designed for one-step synthesis, it provides concise and direct routes for your target compounds, streamlining the synthesis process.
Accurate Predictions: Utilizing the extensive PISTACHIO, BKMS_METABOLIC, PISTACHIO_RINGBREAKER, REAXYS, REAXYS_BIOCATALYSIS database, our tool offers high-accuracy predictions, reflecting the latest in chemical research and data.
Strategy Settings
Precursor scoring | Relevance Heuristic |
---|---|
Min. plausibility | 0.01 |
Model | Template_relevance |
Template Set | Pistachio/Bkms_metabolic/Pistachio_ringbreaker/Reaxys/Reaxys_biocatalysis |
Top-N result to add to graph | 6 |
Feasible Synthetic Routes
Q1: What makes Ethyl Isopropyl Sulfone a promising electrolyte solvent for high-temperature applications in energy storage?
A1: this compound exhibits excellent thermal and electrochemical stability, a crucial characteristic for high-temperature applications. Research shows it enables high capacitance retention in electric double-layer capacitors (EDLCs) even after prolonged exposure to elevated temperatures. For instance, an EDLC utilizing an this compound-based electrolyte retained 68% of its initial capacitance after 500 hours at 60°C and 3.4 V. [] This impressive stability stems from the decomposition products of this compound, which form a passivation layer on the electrode surface, hindering further degradation. []
Q2: Can this compound be utilized in fluorine-free energy storage devices?
A2: Yes, this compound has proven successful in developing entirely fluorine-free lithium-ion capacitors (LICs) suitable for high-temperature operation. [] When combined with lithium bis(oxalato)borate, it forms a novel, non-toxic electrolyte that effectively mitigates aluminum anodic dissolution, a common challenge in these devices. []
Q3: How does this compound compare to conventional electrolytes like organic carbonates in lithium-ion battery applications?
A3: While this compound demonstrates higher oxidative stability compared to organic carbonates, its high viscosity and incompatibility with graphitic anodes pose challenges. [] Research suggests incorporating additives like fluoroethylene carbonate (FEC) or co-solvents like ethylene acetate (EA) can improve the performance of this compound-based electrolytes in graphite-based lithium-ion batteries. [] These findings highlight the ongoing efforts to optimize this compound for broader battery applications.
Q4: How does temperature impact the electrochemical behavior of EDLCs containing this compound-based electrolytes?
A4: Ongoing research investigates the intricate relationship between temperature and the electrochemical behavior of EDLCs utilizing this compound-based electrolytes. [] Understanding this interplay is critical for optimizing device performance and lifespan across various operating temperatures.
Q5: Can this compound be used in combination with other solvents for EDLC electrolytes?
A5: Preliminary research suggests that mixtures of this compound with other solvents, such as acetonitrile, could offer potential benefits for EDLC electrolytes. [] Further investigation is necessary to evaluate the performance and feasibility of such mixtures.
Disclaimer and Information on In-Vitro Research Products
Please be aware that all articles and product information presented on BenchChem are intended solely for informational purposes. The products available for purchase on BenchChem are specifically designed for in-vitro studies, which are conducted outside of living organisms. In-vitro studies, derived from the Latin term "in glass," involve experiments performed in controlled laboratory settings using cells or tissues. It is important to note that these products are not categorized as medicines or drugs, and they have not received approval from the FDA for the prevention, treatment, or cure of any medical condition, ailment, or disease. We must emphasize that any form of bodily introduction of these products into humans or animals is strictly prohibited by law. It is essential to adhere to these guidelines to ensure compliance with legal and ethical standards in research and experimentation.