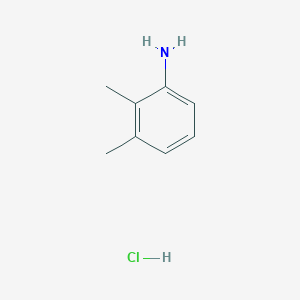
2,3-Dimethylaniline Hydrochloride
Overview
Description
2,3-Dimethylaniline Hydrochloride is an organic compound that belongs to the class of aromatic amines. It is a derivative of aniline, where two methyl groups are substituted at the 2nd and 3rd positions of the benzene ring. This compound is typically found in its hydrochloride salt form, which enhances its solubility in water and other polar solvents. It is used in various chemical syntheses and industrial applications.
Preparation Methods
Synthetic Routes and Reaction Conditions: 2,3-Dimethylaniline Hydrochloride can be synthesized through several methods. One common approach involves the methylation of aniline. The process typically includes the following steps:
Nitration of Toluene: Toluene is nitrated to form 2,3-dimethylnitrobenzene.
Reduction: The nitro group is then reduced to an amine group, resulting in 2,3-dimethylaniline.
Formation of Hydrochloride Salt: The free base 2,3-dimethylaniline is treated with hydrochloric acid to form this compound.
Industrial Production Methods: Industrial production of this compound often involves the catalytic hydrogenation of 2,3-dimethylnitrobenzene in the presence of a suitable catalyst such as palladium on carbon. The resulting 2,3-dimethylaniline is then converted to its hydrochloride salt by reaction with hydrochloric acid.
Chemical Reactions Analysis
Types of Reactions: 2,3-Dimethylaniline Hydrochloride undergoes various chemical reactions, including:
Oxidation: It can be oxidized to form corresponding quinones or other oxidized derivatives.
Reduction: The compound can be reduced further, although this is less common.
Substitution: Electrophilic aromatic substitution reactions are common, where the methyl groups and the amine group direct incoming electrophiles to specific positions on the benzene ring.
Common Reagents and Conditions:
Oxidizing Agents: Potassium permanganate, chromium trioxide.
Reducing Agents: Hydrogen gas with a palladium catalyst.
Substitution Reagents: Halogens, nitrating agents, sulfonating agents.
Major Products:
Oxidation Products: Quinones, nitroso compounds.
Substitution Products: Halogenated derivatives, nitro derivatives, sulfonated derivatives.
Scientific Research Applications
2,3-Dimethylaniline Hydrochloride has several applications in scientific research:
Chemistry: Used as an intermediate in the synthesis of dyes, pigments, and other organic compounds.
Biology: Employed in the study of enzyme interactions and as a reagent in biochemical assays.
Medicine: Investigated for its potential use in the synthesis of pharmaceutical compounds.
Industry: Utilized in the production of polymers, resins, and other industrial chemicals.
Mechanism of Action
The mechanism of action of 2,3-Dimethylaniline Hydrochloride involves its interaction with various molecular targets. As an aromatic amine, it can participate in nucleophilic substitution reactions, where it donates its lone pair of electrons to electrophilic centers. This property makes it useful in the synthesis of complex organic molecules. Additionally, its hydrochloride form enhances its solubility, facilitating its use in aqueous reactions.
Comparison with Similar Compounds
2,4-Dimethylaniline: Another isomer with methyl groups at the 2nd and 4th positions.
2,5-Dimethylaniline: Methyl groups at the 2nd and 5th positions.
2,6-Dimethylaniline: Methyl groups at the 2nd and 6th positions.
3,4-Dimethylaniline: Methyl groups at the 3rd and 4th positions.
3,5-Dimethylaniline: Methyl groups at the 3rd and 5th positions.
Uniqueness: 2,3-Dimethylaniline Hydrochloride is unique due to the specific positioning of its methyl groups, which influences its reactivity and the types of reactions it undergoes. The ortho-substitution pattern (2,3-positions) affects the electron density on the benzene ring, making it distinct from other isomers in terms of chemical behavior and applications.
Properties
IUPAC Name |
2,3-dimethylaniline;hydrochloride | |
---|---|---|
Source | PubChem | |
URL | https://pubchem.ncbi.nlm.nih.gov | |
Description | Data deposited in or computed by PubChem | |
InChI |
InChI=1S/C8H11N.ClH/c1-6-4-3-5-8(9)7(6)2;/h3-5H,9H2,1-2H3;1H | |
Source | PubChem | |
URL | https://pubchem.ncbi.nlm.nih.gov | |
Description | Data deposited in or computed by PubChem | |
InChI Key |
DUQDEEVOZDZJGQ-UHFFFAOYSA-N | |
Source | PubChem | |
URL | https://pubchem.ncbi.nlm.nih.gov | |
Description | Data deposited in or computed by PubChem | |
Canonical SMILES |
CC1=C(C(=CC=C1)N)C.Cl | |
Source | PubChem | |
URL | https://pubchem.ncbi.nlm.nih.gov | |
Description | Data deposited in or computed by PubChem | |
Molecular Formula |
C8H12ClN | |
Source | PubChem | |
URL | https://pubchem.ncbi.nlm.nih.gov | |
Description | Data deposited in or computed by PubChem | |
DSSTOX Substance ID |
DTXSID20988578 | |
Record name | 2,3-Dimethylaniline--hydrogen chloride (1/1) | |
Source | EPA DSSTox | |
URL | https://comptox.epa.gov/dashboard/DTXSID20988578 | |
Description | DSSTox provides a high quality public chemistry resource for supporting improved predictive toxicology. | |
Molecular Weight |
157.64 g/mol | |
Source | PubChem | |
URL | https://pubchem.ncbi.nlm.nih.gov | |
Description | Data deposited in or computed by PubChem | |
CAS No. |
5417-45-8, 68877-30-5 | |
Record name | 5417-45-8 | |
Source | DTP/NCI | |
URL | https://dtp.cancer.gov/dtpstandard/servlet/dwindex?searchtype=NSC&outputformat=html&searchlist=7641 | |
Description | The NCI Development Therapeutics Program (DTP) provides services and resources to the academic and private-sector research communities worldwide to facilitate the discovery and development of new cancer therapeutic agents. | |
Explanation | Unless otherwise indicated, all text within NCI products is free of copyright and may be reused without our permission. Credit the National Cancer Institute as the source. | |
Record name | Benzenamine, ar,ar-dimethyl-, hydrochloride (1:1) | |
Source | EPA Chemicals under the TSCA | |
URL | https://www.epa.gov/chemicals-under-tsca | |
Description | EPA Chemicals under the Toxic Substances Control Act (TSCA) collection contains information on chemicals and their regulations under TSCA, including non-confidential content from the TSCA Chemical Substance Inventory and Chemical Data Reporting. | |
Record name | 2,3-Dimethylaniline--hydrogen chloride (1/1) | |
Source | EPA DSSTox | |
URL | https://comptox.epa.gov/dashboard/DTXSID20988578 | |
Description | DSSTox provides a high quality public chemistry resource for supporting improved predictive toxicology. | |
Retrosynthesis Analysis
AI-Powered Synthesis Planning: Our tool employs the Template_relevance Pistachio, Template_relevance Bkms_metabolic, Template_relevance Pistachio_ringbreaker, Template_relevance Reaxys, Template_relevance Reaxys_biocatalysis model, leveraging a vast database of chemical reactions to predict feasible synthetic routes.
One-Step Synthesis Focus: Specifically designed for one-step synthesis, it provides concise and direct routes for your target compounds, streamlining the synthesis process.
Accurate Predictions: Utilizing the extensive PISTACHIO, BKMS_METABOLIC, PISTACHIO_RINGBREAKER, REAXYS, REAXYS_BIOCATALYSIS database, our tool offers high-accuracy predictions, reflecting the latest in chemical research and data.
Strategy Settings
Precursor scoring | Relevance Heuristic |
---|---|
Min. plausibility | 0.01 |
Model | Template_relevance |
Template Set | Pistachio/Bkms_metabolic/Pistachio_ringbreaker/Reaxys/Reaxys_biocatalysis |
Top-N result to add to graph | 6 |
Feasible Synthetic Routes
Disclaimer and Information on In-Vitro Research Products
Please be aware that all articles and product information presented on BenchChem are intended solely for informational purposes. The products available for purchase on BenchChem are specifically designed for in-vitro studies, which are conducted outside of living organisms. In-vitro studies, derived from the Latin term "in glass," involve experiments performed in controlled laboratory settings using cells or tissues. It is important to note that these products are not categorized as medicines or drugs, and they have not received approval from the FDA for the prevention, treatment, or cure of any medical condition, ailment, or disease. We must emphasize that any form of bodily introduction of these products into humans or animals is strictly prohibited by law. It is essential to adhere to these guidelines to ensure compliance with legal and ethical standards in research and experimentation.