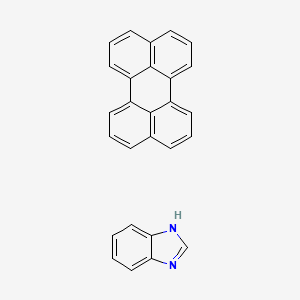
Benzimidazole perylene
Overview
Description
Benzimidazole Perylene is a novel asymmetrically substituted discotic molecule . It was designed with an imidazole unit at 3,4 positions and a bisester moiety at 9,10 positions of the perylene tetracarboxylic acid core . This structure exhibits potentially useful physical properties, including a nonlinear dielectric response to an increasing electric field .
Synthesis Analysis
The synthesis of this compound involves 1,2-aromatic diamines and alkyl or aryl carboxylic acids reacting in electrostatically charged microdroplets . The reactions are accelerated by orders of magnitude in comparison to the bulk . An acid-catalyzed reaction mechanism based on the identification of the intermediate arylamides has been suggested .
Molecular Structure Analysis
The molecular structure of this compound is characterized by a perylene bisbenzimidazole comprising both donor and acceptor functional groups . This structure exhibits potentially useful physical properties, including a nonlinear dielectric response to an increasing electric field .
Chemical Reactions Analysis
The chemical reactions of this compound involve an acid-catalyzed reaction mechanism based on the identification of the intermediate arylamides . Their dehydration to give benzimidazoles occurs in a subsequent thermally enhanced step .
Physical and Chemical Properties Analysis
This compound exhibits potentially useful physical properties, including a nonlinear dielectric response to an increasing electric field . Despite its low surface area, it shows good CO2 uptake and pH responsive behavior owing to the presence of benzimidazole rings .
Scientific Research Applications
Mechanism of Action and Biological Impact of Benzimidazole Compounds :
- Benzimidazole compounds, including those with perylene structures, have been studied extensively for their role as specific inhibitors of microtubule assembly. This is mainly due to their ability to bind to the tubulin molecule. Such studies have significant implications in understanding fungal cell biology and molecular genetics (Davidse, 1986).
Applications in Gas Adsorption and Supercapacitor Electrode Materials :
- Benzimidazole linked to arylimide based covalent organic frameworks, including those with perylene, have been synthesized for special interactions with carbon dioxide. These materials exhibit high surface area and are significant for applications like gas adsorption and supercapacitors (Roy et al., 2017).
Green Synthesis and Biological Functions :
- Benzimidazole moieties, including those integrated with perylene, have a range of applications in pharmaceutical chemistry due to their broad biological functions. These include roles as anti-ulcer, anti-tumor, anti-viral agents, and as DNA minor groove binding agents with significant anti-tumor activity (Kathirvelan et al., 2013).
Liquid Crystalline Perylene Diester Benzimidazoles :
- Studies on perylene diester benzimidazoles have revealed their potential in forming liquid crystalline columnar hexagonal phases, which are significant for applications in materials science due to their extended absorption in the visible regime (Wicklein et al., 2010).
Carrier Generation in Thin Film Structures :
- Research on benzimidazole perylene/tetraphenyldiamine thin film structures has provided insights into carrier generation mechanisms, important for the development of photovoltaic and other electronic devices (Popović et al., 1988).
Electrochemical and Optical Properties for Semiconductors :
- Perylene bis(dicarboximide) and perylene bis(benzimidazole) derivatives have been synthesized as n-type semiconductors, with their electronic properties modulated by the nature of substitution at the bay region. This has applications in optoelectronics and solar energy harvesting (Perrin & Hudhomme, 2011).
Encapsulation in Metal-Azolate Frameworks for Sensing Applications :
- The encapsulation of perylene in zinc benzimidazolate frameworks has been explored to create materials with unique luminescent and fluorescent sensing properties. This is significant in the field of material science and sensing technologies (Fan et al., 2022).
n-Type Field-Effect Transistors :
- This compound has been used in the synthesis of field-effect transistors, demonstrating its utility in advanced electronic devices (Kolhe et al., 2010).
Future Directions
Mechanism of Action
Target of Action
Benzimidazole perylene primarily targets microtubules within cells. Microtubules are essential components of the cytoskeleton, playing a crucial role in maintaining cell shape, enabling intracellular transport, and facilitating cell division. By targeting microtubules, this compound interferes with these critical cellular processes, making it a potent agent in disrupting cell proliferation, particularly in cancer cells .
Mode of Action
This compound interacts with tubulin, the protein subunit of microtubules. This interaction inhibits the polymerization of tubulin into microtubules, leading to the destabilization of the microtubule network. As a result, this compound effectively halts cell division by preventing the formation of the mitotic spindle, a structure necessary for chromosome segregation during mitosis .
Biochemical Pathways
The action of this compound affects several biochemical pathways, primarily those involved in cell cycle regulation. By inhibiting microtubule formation, this compound activates the spindle assembly checkpoint, a safety mechanism that ensures proper chromosome alignment before cell division proceeds. This activation leads to cell cycle arrest at the metaphase stage, preventing the completion of mitosis and ultimately inducing apoptosis, or programmed cell death .
Pharmacokinetics
The pharmacokinetics of this compound involve its absorption, distribution, metabolism, and excretion (ADME) properties. This compound is typically administered orally and is absorbed through the gastrointestinal tract. It exhibits moderate bioavailability due to first-pass metabolism in the liver. The compound is widely distributed throughout the body, with a preference for tissues with high microtubule activity. This compound is metabolized primarily in the liver and excreted via the kidneys. Its half-life and clearance rates are influenced by factors such as liver function and renal health .
Result of Action
The molecular and cellular effects of this compound’s action include the disruption of microtubule dynamics, leading to cell cycle arrest and apoptosis. At the molecular level, this compound binds to the colchicine-binding site on tubulin, preventing its polymerization. This binding disrupts the normal function of microtubules, leading to the collapse of the mitotic spindle and subsequent cell death. These effects are particularly pronounced in rapidly dividing cells, such as cancer cells, making this compound a valuable chemotherapeutic agent .
Action Environment
Environmental factors can significantly influence the action, efficacy, and stability of this compound. Factors such as pH, temperature, and the presence of other chemicals can affect the compound’s stability and bioavailability. For instance, acidic environments may enhance the solubility of this compound, improving its absorption in the gastrointestinal tract. Conversely, extreme temperatures or the presence of reactive chemicals may degrade the compound, reducing its efficacy. Understanding these environmental influences is crucial for optimizing the therapeutic use of this compound .
: Springer : DrugBank : Cell Press
Properties
IUPAC Name |
1H-benzimidazole;perylene | |
---|---|---|
Source | PubChem | |
URL | https://pubchem.ncbi.nlm.nih.gov | |
Description | Data deposited in or computed by PubChem | |
InChI |
InChI=1S/C20H12.C7H6N2/c1-5-13-6-2-11-17-18-12-4-8-14-7-3-10-16(20(14)18)15(9-1)19(13)17;1-2-4-7-6(3-1)8-5-9-7/h1-12H;1-5H,(H,8,9) | |
Source | PubChem | |
URL | https://pubchem.ncbi.nlm.nih.gov | |
Description | Data deposited in or computed by PubChem | |
InChI Key |
KIIFVSJBFGYDFV-UHFFFAOYSA-N | |
Source | PubChem | |
URL | https://pubchem.ncbi.nlm.nih.gov | |
Description | Data deposited in or computed by PubChem | |
Canonical SMILES |
C1=CC=C2C(=C1)NC=N2.C1=CC2=C3C(=C1)C4=CC=CC5=C4C(=CC=C5)C3=CC=C2 | |
Source | PubChem | |
URL | https://pubchem.ncbi.nlm.nih.gov | |
Description | Data deposited in or computed by PubChem | |
Molecular Formula |
C27H18N2 | |
Source | PubChem | |
URL | https://pubchem.ncbi.nlm.nih.gov | |
Description | Data deposited in or computed by PubChem | |
DSSTOX Substance ID |
DTXSID80619952 | |
Record name | 1H-Benzimidazole--perylene (1/1) | |
Source | EPA DSSTox | |
URL | https://comptox.epa.gov/dashboard/DTXSID80619952 | |
Description | DSSTox provides a high quality public chemistry resource for supporting improved predictive toxicology. | |
Molecular Weight |
370.4 g/mol | |
Source | PubChem | |
URL | https://pubchem.ncbi.nlm.nih.gov | |
Description | Data deposited in or computed by PubChem | |
CAS No. |
79534-91-1 | |
Record name | 1H-Benzimidazole--perylene (1/1) | |
Source | EPA DSSTox | |
URL | https://comptox.epa.gov/dashboard/DTXSID80619952 | |
Description | DSSTox provides a high quality public chemistry resource for supporting improved predictive toxicology. | |
Synthesis routes and methods I
Procedure details
Synthesis routes and methods II
Procedure details
Retrosynthesis Analysis
AI-Powered Synthesis Planning: Our tool employs the Template_relevance Pistachio, Template_relevance Bkms_metabolic, Template_relevance Pistachio_ringbreaker, Template_relevance Reaxys, Template_relevance Reaxys_biocatalysis model, leveraging a vast database of chemical reactions to predict feasible synthetic routes.
One-Step Synthesis Focus: Specifically designed for one-step synthesis, it provides concise and direct routes for your target compounds, streamlining the synthesis process.
Accurate Predictions: Utilizing the extensive PISTACHIO, BKMS_METABOLIC, PISTACHIO_RINGBREAKER, REAXYS, REAXYS_BIOCATALYSIS database, our tool offers high-accuracy predictions, reflecting the latest in chemical research and data.
Strategy Settings
Precursor scoring | Relevance Heuristic |
---|---|
Min. plausibility | 0.01 |
Model | Template_relevance |
Template Set | Pistachio/Bkms_metabolic/Pistachio_ringbreaker/Reaxys/Reaxys_biocatalysis |
Top-N result to add to graph | 6 |
Feasible Synthetic Routes
Q1: What are the typical applications of benzimidazole perylene (BZP)?
A1: this compound (BZP) is primarily known for its excellent light-absorbing properties, making it a suitable candidate for applications in organic electronics. Research shows its effectiveness as a photogenerator material in image sensor arrays and xerographic photoreceptors. [, ].
Q2: How does BZP perform as a photogenerator material compared to other organic pigments?
A2: Studies have demonstrated that BZP, when used in evaporated thin film form, exhibits superior carrier generation efficiency compared to its particle dispersion form in xerographic photoreceptors. Specifically, BZP evaporated films achieved almost double the efficiency. []
Q3: What causes the difference in carrier generation efficiency between BZP thin films and particle dispersions?
A3: Time-resolved fluorescence measurements suggest that the reduced efficiency in particle dispersions stems from exciton bulk trapping. This phenomenon limits the number of excitons reaching the interface between BZP and the hole transport layer (typically tetraphenyldiamine, TPD), hindering efficient carrier generation which relies on exciton dissociation at this interface. []
Q4: Can you elaborate on the role of TPD in devices utilizing BZP?
A4: Tetraphenyldiamine (TPD) acts as a hole transport layer in conjunction with BZP. The BZP/TPD interface is crucial for carrier generation, where excitons dissociate into electron-hole pairs. The TPD layer then facilitates the transport of holes generated during this process. [, ]
Q5: Are there methods to improve the solubility of BZP for processing and device fabrication?
A5: Yes, a Lewis acid pigment solubilization (LAPS) process has been developed to enhance the solubility of BZP and other heterocyclic pigments. This method utilizes a Lewis acid, such as aluminum chloride, in nitromethane to dissolve BZP, facilitating the fabrication of thin film devices. []
Q6: Are there known structural differences between different forms of BZP?
A7: Yes, BZP exists in both cis and trans forms. Both forms have been structurally characterized using X-ray crystallography. The trans form exhibits a planar structure with a center of symmetry, while the cis form, although planar, lacks a center of symmetry due to orientational disorder. [, ]
Q7: Have any modifications been made to the BZP structure to improve its properties?
A8: Yes, researchers have synthesized novel bridged double this compound derivatives and studied their optical properties and film morphology [, ]. Additionally, the introduction of perfluoroalkyl substituents to the BZP core has been explored to enhance solubility, thermal stability, and overall stability [].
Disclaimer and Information on In-Vitro Research Products
Please be aware that all articles and product information presented on BenchChem are intended solely for informational purposes. The products available for purchase on BenchChem are specifically designed for in-vitro studies, which are conducted outside of living organisms. In-vitro studies, derived from the Latin term "in glass," involve experiments performed in controlled laboratory settings using cells or tissues. It is important to note that these products are not categorized as medicines or drugs, and they have not received approval from the FDA for the prevention, treatment, or cure of any medical condition, ailment, or disease. We must emphasize that any form of bodily introduction of these products into humans or animals is strictly prohibited by law. It is essential to adhere to these guidelines to ensure compliance with legal and ethical standards in research and experimentation.