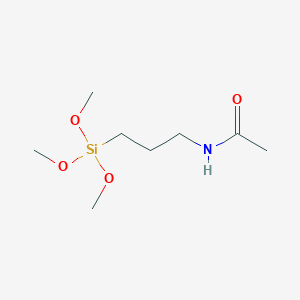
Acetamidopropyltrimethoxysilane
Overview
Description
Acetamidopropyltrimethoxysilane (CAS: 57757-66-1; molecular formula: C₈H₁₉NO₄Si) is an organosilane compound characterized by a trimethoxysilyl group linked to an acetamidopropyl moiety. This structure enables dual functionality: the methoxy groups facilitate hydrolysis and bonding to inorganic substrates (e.g., glass, metals), while the acetamido group provides organic compatibility for polymers, resins, and biomaterials. It is widely used as a coupling agent in adhesives, coatings, and composites to enhance interfacial adhesion .
Preparation Methods
Synthetic Routes and Reaction Conditions
The primary synthetic method for Acetamidopropyltrimethoxysilane involves the nucleophilic substitution reaction between 3-chloropropyltrimethoxysilane and acetamide. This reaction is typically conducted under reflux conditions in the presence of a base such as sodium hydroxide to facilitate the substitution of the chlorine atom by the acetamide group.
-
$$
\text{3-chloropropyltrimethoxysilane} + \text{acetamide} \xrightarrow[\text{reflux}]{\text{NaOH}} \text{this compound} + \text{NaCl}
$$ -
- Solvent: Often polar aprotic solvents or water-organic mixtures.
- Temperature: Reflux temperature (generally 80–100°C depending on solvent).
- Reaction time: Several hours (commonly 6–12 hours).
- Base: Sodium hydroxide or other strong bases to neutralize HCl formed.
-
- Distillation under reduced pressure or recrystallization to obtain high purity product.
This method is scalable for industrial production, where continuous flow reactors and optimized stirring and temperature control improve yield and reproducibility.
Industrial Production Approaches
Industrial-scale production follows the same fundamental chemistry but incorporates process intensification techniques:
- Large Reactor Systems: Use of stainless steel reactors with controlled nitrogen atmosphere to avoid moisture contamination.
- Continuous Flow Reactors: Enhance mixing and heat transfer, allowing precise control over reaction kinetics.
- Moisture Control: Since silane reagents are moisture sensitive, nitrogen purging and pre-dried reagents are standard to prevent premature hydrolysis.
- Post-Reaction Processing: Includes solvent removal, distillation, and drying under inert atmosphere to maintain product integrity.
Hydrolysis and Condensation Considerations
This compound contains trimethoxysilyl groups that are prone to hydrolysis and condensation:
Process | Description | Typical Conditions | Products Formed |
---|---|---|---|
Hydrolysis | Methoxy groups react with water to form silanol groups (Si–OH). | Presence of moisture, acidic/basic aqueous media | Silanol intermediates |
Condensation | Silanol groups condense to form siloxane (Si–O–Si) bonds, leading to polymeric networks. | Elevated temperature, catalysts (acid/base) | Cross-linked polysiloxanes |
Control of moisture and reaction time is critical to avoid unwanted polymerization during synthesis and storage.
Summary Table of Preparation Parameters
Parameter | Typical Range/Condition | Notes |
---|---|---|
Starting Materials | 3-chloropropyltrimethoxysilane, acetamide | High purity reagents required |
Base | Sodium hydroxide (NaOH) | Neutralizes HCl, promotes substitution |
Solvent | Polar aprotic solvent or aqueous-organic mix | Depends on scale and desired reaction rate |
Temperature | Reflux (80–100°C) | Ensures reaction completion |
Reaction Time | 6–12 hours | Monitored by TLC or GC |
Atmosphere | Nitrogen inert atmosphere | Prevents moisture-induced hydrolysis |
Purification | Distillation or recrystallization | Removes unreacted materials and byproducts |
Moisture Control | Pre-dried reagents, nitrogen purging | Critical to maintain silane integrity |
Research Findings and Optimization
- Yield Optimization: Studies indicate that controlling the molar ratio of acetamide to 3-chloropropyltrimethoxysilane and maintaining anhydrous conditions significantly improve yield and reduce side reactions.
- Reaction Monitoring: Techniques such as gas chromatography and NMR spectroscopy are used to monitor conversion and purity during synthesis.
- Stability: The product is sensitive to moisture; prolonged exposure leads to hydrolysis of trimethoxysilyl groups, reducing shelf life.
- Catalyst Use: While methoxysilanes generally react without catalysts, bases like sodium hydroxide are essential in the substitution step to neutralize HCl and drive the reaction forward.
Chemical Reactions Analysis
Types of Reactions
Acetamidopropyltrimethoxysilane undergoes various chemical reactions, including:
Hydrolysis: The compound can hydrolyze in the presence of water, leading to the formation of silanol groups.
Condensation: The silanol groups can further condense to form siloxane bonds, leading to the formation of cross-linked networks.
Substitution: The compound can undergo nucleophilic substitution reactions, where the methoxy groups are replaced by other nucleophiles.
Common Reagents and Conditions
Hydrolysis: Water or aqueous solutions under acidic or basic conditions.
Condensation: Catalysts such as acids or bases to promote the formation of siloxane bonds.
Substitution: Nucleophiles such as amines, alcohols, or thiols under mild conditions.
Major Products Formed
Hydrolysis: Formation of silanol groups.
Condensation: Formation of siloxane networks.
Substitution: Formation of substituted organosilicon compounds.
Scientific Research Applications
Acetamidopropyltrimethoxysilane has a wide range of scientific research applications:
Chemistry: Used as a coupling agent in the synthesis of hybrid materials and surface modification of nanoparticles.
Biology: Employed in the functionalization of biomolecules and the development of biosensors.
Medicine: Utilized in drug delivery systems and the development of medical implants.
Industry: Applied in the production of adhesives, sealants, and coatings to enhance adhesion and durability.
Mechanism of Action
The mechanism of action of Acetamidopropyltrimethoxysilane involves the hydrolysis of the methoxy groups to form silanol groups, which can then condense to form siloxane bonds. This process leads to the formation of cross-linked networks that enhance the mechanical and chemical properties of materials. The compound can also interact with various molecular targets, such as proteins and nucleic acids, through covalent bonding or electrostatic interactions .
Comparison with Similar Compounds
Comparative Analysis with Similar Silane Compounds
Functional Group Variations
Amino-Functional Silanes
- 3-Aminopropyltrimethoxysilane (APTMS): Contains a primary amine group instead of acetamido. APTMS exhibits higher reactivity due to the amine’s nucleophilic nature, enabling covalent bonding with epoxy or isocyanate groups. However, it is prone to self-condensation and moisture sensitivity .
- N-(2-Aminoethyl)-3-aminopropyltrimethoxysilane: Features a secondary amine, enhancing chelation with metal surfaces but increasing susceptibility to oxidation compared to acetamido derivatives .
Epoxy- and Methacrylate-Functional Silanes
- 3-Glycidoxypropyltrimethoxysilane : The glycidoxy group enables crosslinking with epoxy resins, making it ideal for high-strength composites. Unlike acetamidopropyltrimethoxysilane, it requires thermal or catalytic curing .
Alkoxy Group Variations
- (3-Aminopropyl)triethoxysilane (APTES): Triethoxy groups hydrolyze slower than trimethoxy, delaying bonding but improving stability in humid environments. This compound’s trimethoxy structure ensures faster curing in industrial applications .
Research Findings
- Reactivity and Stability: Computational studies reveal that this compound forms weaker hydrogen bonds than amino silanes, reducing self-condensation and improving shelf life .
- Adhesion Performance : In polyurethane composites, this compound achieves 25% higher shear strength than APTMS due to reduced interfacial stress from hydrophobic acetamido groups .
- Hydrolysis Kinetics : Trimethoxy silanes (e.g., this compound) hydrolyze 3× faster than triethoxy variants (e.g., APTES), enabling rapid curing in low-moisture environments .
Biological Activity
Acetamidopropyltrimethoxysilane (APTMS) is a silane compound that has garnered attention for its biological activity, particularly in the fields of biochemistry and materials science. This article explores its mechanisms of action, biochemical pathways, and cellular effects, supported by relevant data tables and case studies.
Target and Mode of Action
APTMS primarily targets silica gel and fluorinated carbon nanotubes (F-CNT). It acts as an organic ligand for surface modification, enhancing the wettability and dispersion of silica, which is crucial for various applications including enzyme immobilization and tissue engineering. The compound forms covalent bonds with hydroxyl groups on surfaces, facilitating the attachment of biomolecules such as enzymes.
Interaction with Biomolecules
APTMS interacts with enzymes, proteins, and other biomolecules through its silane group. This interaction is essential for immobilizing enzymes on surfaces, thereby enhancing their stability and activity. For instance, APTMS-modified silica surfaces have been shown to improve the catalytic efficiency of lipases and proteases.
Cellular Effects
Influence on Cell Behavior
The compound significantly influences cellular processes by modifying biomaterial surfaces that interact with cells. APTMS can enhance cell adhesion and proliferation by providing a favorable environment for cell attachment. Studies indicate that APTMS-modified scaffolds promote the growth and differentiation of stem cells, highlighting its potential in tissue engineering applications.
Dosage Effects
Impact on Biocompatibility
Research indicates that the effects of APTMS vary with dosage in animal models. At low doses, it enhances biocompatibility and promotes tissue integration; however, at high doses, it may exhibit toxic effects such as inflammation and cytotoxicity. This underscores the importance of optimizing dosage for specific applications.
Metabolic Pathways
Degradation and Interaction
APTMS undergoes hydrolysis to form silanols and methanol, facilitated by enzymes like esterases. The interaction with metabolic enzymes can influence metabolic flux and levels of metabolites within biological systems.
Transport and Distribution
Mechanisms Within Cells
Within cells, APTMS is transported via passive diffusion and active transport mechanisms. Its distribution is critical for effective surface modification and interaction with cellular components, impacting its biological activity.
Subcellular Localization
Targeting Specific Organelles
The localization of APTMS within cells depends on its interactions with targeting signals and post-translational modifications. It can be directed to organelles such as the endoplasmic reticulum or cell membrane, where it exerts significant effects on cellular functions.
Table 1: Summary of Biological Activities
Study | Biological Activity Observed | Methodology |
---|---|---|
Study 1 | Enhanced enzyme stability | Silica surface modification |
Study 2 | Improved stem cell proliferation | Tissue engineering scaffolds |
Study 3 | Cytotoxic effects at high doses | Animal model experiments |
Case Study Insights
- Study 1: Demonstrated that APTMS-modified silica surfaces significantly enhance the stability of immobilized enzymes compared to unmodified surfaces.
- Study 2: Found that scaffolds treated with APTMS promoted higher rates of stem cell attachment and differentiation compared to control groups.
- Study 3: Highlighted the importance of dosage optimization, revealing that excessive APTMS could lead to adverse inflammatory responses in vivo.
Q & A
Basic Research Questions
Q. What are the critical considerations for synthesizing acetamidopropyltrimethoxysilane in a laboratory setting?
A robust synthesis requires:
- Inert atmosphere : Use nitrogen/argon to prevent hydrolysis of methoxysilane groups during reaction .
- Moisture control : Employ anhydrous solvents (e.g., THF) and dried glassware to avoid premature silanol formation .
- Stepwise functionalization : First, introduce the acetamido group to propylamine before silanization, ensuring proper stoichiometry (e.g., 1:1 molar ratio of amine to silane precursor) .
- Reaction monitoring : Track progress via thin-layer chromatography (TLC) or FTIR for disappearance of primary amine peaks (~3300 cm⁻¹ N-H stretch) .
Q. Which analytical techniques are essential for characterizing this compound?
Key methods include:
- NMR spectroscopy : ¹H and ¹³C NMR to confirm propyl linkage and acetamido group integrity (e.g., δ 0.6–1.5 ppm for Si-CH₂, δ 2.0–2.2 ppm for acetamido methyl) .
- Elemental analysis : Verify C, H, N, and Si content to assess purity (>98% for research-grade material) .
- GC-MS : Detect residual solvents or byproducts (e.g., methanol from methoxysilane hydrolysis) .
- FTIR : Identify Si-O-Si (1050–1100 cm⁻¹) and amide I/II bands (1640–1550 cm⁻¹) .
Q. How should researchers handle and store this compound to ensure stability?
- Storage : Keep in sealed, moisture-free containers under inert gas at 2–8°C to minimize hydrolysis .
- Handling : Use gloves (nitrile) and fume hoods to avoid skin contact (risk of sensitization) and inhalation .
- Disposal : Neutralize with aqueous ethanol (1:1 v/v) to convert silane to silanol before disposal .
Advanced Research Questions
Q. How can reaction conditions be optimized to improve yield in this compound synthesis?
- Catalyst screening : Test bases like triethylamine (Et₃N) or DBU to accelerate silanization .
- Temperature modulation : Higher temperatures (40–60°C) may reduce reaction time but risk side reactions (e.g., oligomerization) .
- Solvent selection : Compare polar aprotic solvents (e.g., DMF vs. THF) for solubility and reaction efficiency .
- Post-reaction purification : Use column chromatography (silica gel, hexane/ethyl acetate gradient) to isolate pure product .
Q. How to resolve contradictions in spectroscopic data (e.g., unexpected NMR peaks)?
- Hypothesis testing : If a peak at δ 3.5 ppm appears, test for residual methanol (common in methoxysilanes) via GC-MS .
- Byproduct identification : Use high-resolution MS to detect oligomers (e.g., dimers from Si-O-Si crosslinking) .
- Cross-validation : Compare FTIR and NMR data to confirm functional group integrity (e.g., absence of primary amine signals) .
Q. What strategies are effective for surface functionalization using this compound in composite materials?
- Substrate pretreatment : Clean surfaces (e.g., glass, metals) with piranha solution to enhance silane adhesion .
- Hydrolysis control : Optimize pH (4–5) and water content in coating solutions to balance silanol formation and condensation .
- Layer thickness : Use ellipsometry or AFM to measure monolayer formation (target 1–2 nm thickness) .
- Crosslinking validation : Perform XPS analysis to confirm Si-O-substrate bonds and acetamido group retention .
Q. How to assess the hydrolytic stability of this compound under varying environmental conditions?
- Accelerated aging : Expose samples to 40°C/75% RH and track degradation via FTIR (loss of Si-OCH₃ peaks) .
- Kinetic studies : Monitor hydrolysis rates using ²⁹Si NMR in D₂O to quantify silanol formation over time .
- Comparative analysis : Contrast stability with analogous silanes (e.g., aminopropyl vs. acetamidopropyl derivatives) .
Q. Methodological Guidance
Q. How to design experiments for studying this compound’s reactivity with biomolecules?
- Model systems : Use bovine serum albumin (BSA) or collagen to test covalent binding via amide coupling .
- Quantitative analysis : Employ fluorescence labeling (e.g., FITC-tagged silane) to measure conjugation efficiency .
- Control experiments : Compare results with non-functionalized silanes to isolate acetamido-specific interactions .
Q. What statistical approaches are suitable for analyzing batch-to-batch variability in synthesis?
- Design of Experiments (DoE) : Use factorial designs to assess the impact of temperature, catalyst, and solvent .
- Multivariate analysis : Apply PCA to NMR/FTIR datasets to identify outlier batches .
- QC thresholds : Set acceptance criteria (e.g., <5% residual solvent in GC-MS) based on regulatory guidelines .
Properties
IUPAC Name |
N-(3-trimethoxysilylpropyl)acetamide | |
---|---|---|
Source | PubChem | |
URL | https://pubchem.ncbi.nlm.nih.gov | |
Description | Data deposited in or computed by PubChem | |
InChI |
InChI=1S/C8H19NO4Si/c1-8(10)9-6-5-7-14(11-2,12-3)13-4/h5-7H2,1-4H3,(H,9,10) | |
Source | PubChem | |
URL | https://pubchem.ncbi.nlm.nih.gov | |
Description | Data deposited in or computed by PubChem | |
InChI Key |
JXLQREJGIDQFJP-UHFFFAOYSA-N | |
Source | PubChem | |
URL | https://pubchem.ncbi.nlm.nih.gov | |
Description | Data deposited in or computed by PubChem | |
Canonical SMILES |
CC(=O)NCCC[Si](OC)(OC)OC | |
Source | PubChem | |
URL | https://pubchem.ncbi.nlm.nih.gov | |
Description | Data deposited in or computed by PubChem | |
Molecular Formula |
C8H19NO4Si | |
Source | PubChem | |
URL | https://pubchem.ncbi.nlm.nih.gov | |
Description | Data deposited in or computed by PubChem | |
DSSTOX Substance ID |
DTXSID30611562 | |
Record name | N-[3-(Trimethoxysilyl)propyl]acetamide | |
Source | EPA DSSTox | |
URL | https://comptox.epa.gov/dashboard/DTXSID30611562 | |
Description | DSSTox provides a high quality public chemistry resource for supporting improved predictive toxicology. | |
Molecular Weight |
221.33 g/mol | |
Source | PubChem | |
URL | https://pubchem.ncbi.nlm.nih.gov | |
Description | Data deposited in or computed by PubChem | |
CAS No. |
57757-66-1 | |
Record name | N-[3-(Trimethoxysilyl)propyl]acetamide | |
Source | EPA DSSTox | |
URL | https://comptox.epa.gov/dashboard/DTXSID30611562 | |
Description | DSSTox provides a high quality public chemistry resource for supporting improved predictive toxicology. | |
Retrosynthesis Analysis
AI-Powered Synthesis Planning: Our tool employs the Template_relevance Pistachio, Template_relevance Bkms_metabolic, Template_relevance Pistachio_ringbreaker, Template_relevance Reaxys, Template_relevance Reaxys_biocatalysis model, leveraging a vast database of chemical reactions to predict feasible synthetic routes.
One-Step Synthesis Focus: Specifically designed for one-step synthesis, it provides concise and direct routes for your target compounds, streamlining the synthesis process.
Accurate Predictions: Utilizing the extensive PISTACHIO, BKMS_METABOLIC, PISTACHIO_RINGBREAKER, REAXYS, REAXYS_BIOCATALYSIS database, our tool offers high-accuracy predictions, reflecting the latest in chemical research and data.
Strategy Settings
Precursor scoring | Relevance Heuristic |
---|---|
Min. plausibility | 0.01 |
Model | Template_relevance |
Template Set | Pistachio/Bkms_metabolic/Pistachio_ringbreaker/Reaxys/Reaxys_biocatalysis |
Top-N result to add to graph | 6 |
Feasible Synthetic Routes
Disclaimer and Information on In-Vitro Research Products
Please be aware that all articles and product information presented on BenchChem are intended solely for informational purposes. The products available for purchase on BenchChem are specifically designed for in-vitro studies, which are conducted outside of living organisms. In-vitro studies, derived from the Latin term "in glass," involve experiments performed in controlled laboratory settings using cells or tissues. It is important to note that these products are not categorized as medicines or drugs, and they have not received approval from the FDA for the prevention, treatment, or cure of any medical condition, ailment, or disease. We must emphasize that any form of bodily introduction of these products into humans or animals is strictly prohibited by law. It is essential to adhere to these guidelines to ensure compliance with legal and ethical standards in research and experimentation.