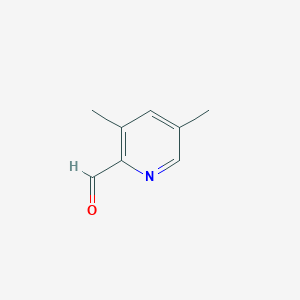
3,5-Dimethylpyridine-2-carboxaldehyde
Overview
Description
3,5-Dimethylpyridine-2-carboxaldehyde (3,5-DMP-2-CHO) is an organic compound that belongs to the family of pyridines, which are aromatic heterocyclic compounds containing six carbon atoms and one nitrogen atom . It is a versatile reagent used in a wide range of applications, such as synthesis, analysis, and biochemistry. It is a colorless liquid with a characteristic odor and is most commonly used as a starting material for the synthesis of other compounds.
Synthesis Analysis
The reaction of 3,5-Dimethylpyridine-2-carboxaldehyde derivatives under grind-stone chemistry conditions has led to the synthesis of novel 1,4-dihydropyridine-3,5-dicarbohydrazone derivatives. Research has explored the green synthesis of various derivatives involving 3,5-Dimethylpyridine-2-carboxaldehyde, emphasizing environmentally friendly approaches to chemical synthesis.Molecular Structure Analysis
The molecular formula of 3,5-Dimethylpyridine-2-carboxaldehyde is C8H9NO . The InChI Key is BYDYILQCRDXHLB-UHFFFAOYSA-N .Chemical Reactions Analysis
3,5-Dimethylpyridine-2-carboxaldehyde is used in a wide range of applications, such as synthesis, analysis, and biochemistry. It is most commonly used as a starting material for the synthesis of other compounds.Physical And Chemical Properties Analysis
3,5-Dimethylpyridine-2-carboxaldehyde is a colorless liquid with a characteristic odor. It has a molecular weight of 135.16 g/mol. The storage temperature is 2-8°C .Scientific Research Applications
Organic Synthesis
3,5-Dimethylpyridine-2-carboxaldehyde: is a valuable intermediate in organic synthesis. It serves as a precursor for the synthesis of various heterocyclic compounds . Its aldehyde group can undergo various chemical reactions, such as condensation, to form Schiff bases, which are useful in synthesizing pharmaceuticals and agrochemicals.
Ligand Design
In coordination chemistry, 3,5-Dimethylpyridine-2-carboxaldehyde can be used to design ligands. These ligands can chelate metal ions, forming complexes with potential catalytic activities for industrial processes . The steric and electronic properties of the ligands can be fine-tuned by modifying the pyridine ring.
Material Science
This compound finds applications in material science, particularly in the development of organic light-emitting diodes (OLEDs) and other electronic materials . Its ability to conduct electricity and emit light when subjected to an electric current makes it a candidate for creating advanced materials.
Analytical Chemistry
3,5-Dimethylpyridine-2-carboxaldehyde: can be utilized as a derivatization agent in analytical chemistry . It reacts with various analytes, enhancing their detection and quantification via spectroscopic methods such as UV-Vis and fluorescence spectroscopy.
Pharmaceutical Research
In pharmaceutical research, this compound is explored for its potential as a building block for drug design . Its structural motif is present in many bioactive molecules, and modifications to the pyridine ring can lead to new pharmacologically active compounds.
Agrochemical Development
The agricultural sector benefits from the use of 3,5-Dimethylpyridine-2-carboxaldehyde in developing new pesticides and herbicides . Its chemical structure can be incorporated into molecules that interact with specific biological targets in pests and weeds, providing effective crop protection.
Mechanism of Action
The reaction of 3,5-Dimethylpyridine-2-carboxaldehyde derivatives under grind-stone chemistry conditions has led to the synthesis of novel 1,4-dihydropyridine-3,5-dicarbohydrazone derivatives. Some of these compounds exhibit promising IC50 values against HepG2 cell lines, indicating their potential anticancer activity.
Safety and Hazards
properties
IUPAC Name |
3,5-dimethylpyridine-2-carbaldehyde | |
---|---|---|
Source | PubChem | |
URL | https://pubchem.ncbi.nlm.nih.gov | |
Description | Data deposited in or computed by PubChem | |
InChI |
InChI=1S/C8H9NO/c1-6-3-7(2)8(5-10)9-4-6/h3-5H,1-2H3 | |
Source | PubChem | |
URL | https://pubchem.ncbi.nlm.nih.gov | |
Description | Data deposited in or computed by PubChem | |
InChI Key |
BYDYILQCRDXHLB-UHFFFAOYSA-N | |
Source | PubChem | |
URL | https://pubchem.ncbi.nlm.nih.gov | |
Description | Data deposited in or computed by PubChem | |
Canonical SMILES |
CC1=CC(=C(N=C1)C=O)C | |
Source | PubChem | |
URL | https://pubchem.ncbi.nlm.nih.gov | |
Description | Data deposited in or computed by PubChem | |
Molecular Formula |
C8H9NO | |
Source | PubChem | |
URL | https://pubchem.ncbi.nlm.nih.gov | |
Description | Data deposited in or computed by PubChem | |
DSSTOX Substance ID |
DTXSID80595883 | |
Record name | 3,5-Dimethylpyridine-2-carbaldehyde | |
Source | EPA DSSTox | |
URL | https://comptox.epa.gov/dashboard/DTXSID80595883 | |
Description | DSSTox provides a high quality public chemistry resource for supporting improved predictive toxicology. | |
Molecular Weight |
135.16 g/mol | |
Source | PubChem | |
URL | https://pubchem.ncbi.nlm.nih.gov | |
Description | Data deposited in or computed by PubChem | |
Product Name |
3,5-Dimethylpyridine-2-carboxaldehyde | |
CAS RN |
675138-02-0 | |
Record name | 3,5-Dimethylpyridine-2-carbaldehyde | |
Source | EPA DSSTox | |
URL | https://comptox.epa.gov/dashboard/DTXSID80595883 | |
Description | DSSTox provides a high quality public chemistry resource for supporting improved predictive toxicology. | |
Record name | 3,5-dimethylpyridine-2-carbaldehyde | |
Source | European Chemicals Agency (ECHA) | |
URL | https://echa.europa.eu/information-on-chemicals | |
Description | The European Chemicals Agency (ECHA) is an agency of the European Union which is the driving force among regulatory authorities in implementing the EU's groundbreaking chemicals legislation for the benefit of human health and the environment as well as for innovation and competitiveness. | |
Explanation | Use of the information, documents and data from the ECHA website is subject to the terms and conditions of this Legal Notice, and subject to other binding limitations provided for under applicable law, the information, documents and data made available on the ECHA website may be reproduced, distributed and/or used, totally or in part, for non-commercial purposes provided that ECHA is acknowledged as the source: "Source: European Chemicals Agency, http://echa.europa.eu/". Such acknowledgement must be included in each copy of the material. ECHA permits and encourages organisations and individuals to create links to the ECHA website under the following cumulative conditions: Links can only be made to webpages that provide a link to the Legal Notice page. | |
Synthesis routes and methods I
Procedure details
Synthesis routes and methods II
Procedure details
Synthesis routes and methods III
Procedure details
Synthesis routes and methods IV
Procedure details
Retrosynthesis Analysis
AI-Powered Synthesis Planning: Our tool employs the Template_relevance Pistachio, Template_relevance Bkms_metabolic, Template_relevance Pistachio_ringbreaker, Template_relevance Reaxys, Template_relevance Reaxys_biocatalysis model, leveraging a vast database of chemical reactions to predict feasible synthetic routes.
One-Step Synthesis Focus: Specifically designed for one-step synthesis, it provides concise and direct routes for your target compounds, streamlining the synthesis process.
Accurate Predictions: Utilizing the extensive PISTACHIO, BKMS_METABOLIC, PISTACHIO_RINGBREAKER, REAXYS, REAXYS_BIOCATALYSIS database, our tool offers high-accuracy predictions, reflecting the latest in chemical research and data.
Strategy Settings
Precursor scoring | Relevance Heuristic |
---|---|
Min. plausibility | 0.01 |
Model | Template_relevance |
Template Set | Pistachio/Bkms_metabolic/Pistachio_ringbreaker/Reaxys/Reaxys_biocatalysis |
Top-N result to add to graph | 6 |
Feasible Synthetic Routes
Disclaimer and Information on In-Vitro Research Products
Please be aware that all articles and product information presented on BenchChem are intended solely for informational purposes. The products available for purchase on BenchChem are specifically designed for in-vitro studies, which are conducted outside of living organisms. In-vitro studies, derived from the Latin term "in glass," involve experiments performed in controlled laboratory settings using cells or tissues. It is important to note that these products are not categorized as medicines or drugs, and they have not received approval from the FDA for the prevention, treatment, or cure of any medical condition, ailment, or disease. We must emphasize that any form of bodily introduction of these products into humans or animals is strictly prohibited by law. It is essential to adhere to these guidelines to ensure compliance with legal and ethical standards in research and experimentation.