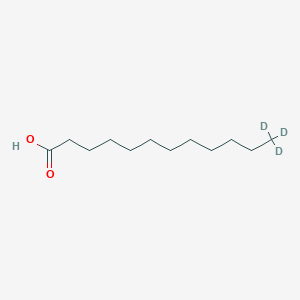
12,12,12-trideuteriododecanoic acid
Overview
Description
12,12,12-trideuteriododecanoic acid: is a deuterium-labeled analogue of dodecanoic acid, also known as lauric acid. This compound is a medium-chain saturated fatty acid with a 12-carbon atom chain, where three hydrogen atoms at the terminal carbon are replaced by deuterium atoms. It is commonly used in scientific research due to its unique properties and applications .
Preparation Methods
Synthetic Routes and Reaction Conditions: The synthesis of 12,12,12-trideuteriododecanoic acid typically involves the deuteration of dodecanoic acid. This process can be achieved through catalytic hydrogenation using deuterium gas (D2) in the presence of a suitable catalyst such as palladium on carbon (Pd/C). The reaction is carried out under controlled temperature and pressure conditions to ensure the selective incorporation of deuterium atoms at the terminal carbon .
Industrial Production Methods: Industrial production of this compound follows similar principles as laboratory synthesis but on a larger scale. The process involves the use of high-pressure reactors and continuous flow systems to achieve efficient deuteration. The purity and yield of the final product are optimized through purification techniques such as distillation and recrystallization .
Chemical Reactions Analysis
Acid-Base Reactions
The carboxylic acid group undergoes typical acid-base reactions. Deuteration at the acidic hydrogen site (C-12) significantly affects proton transfer kinetics due to the primary kinetic isotope effect (KIE).
-
Neutralization : Reacts with bases (e.g., NaOH) to form deuterated carboxylate salts (C<sub>11</sub>H<sub>21</sub>COO<sup>-</sup>Na<sup>+</sup>). The reaction is slower compared to non-deuterated analogs due to reduced acidity (pK<sub>a</sub> increases by ~0.5 units) .
Esterification
Deuterium substitution influences esterification with alcohols under acidic catalysis. The C-12 deuterium reduces the rate of nucleophilic attack at the carbonyl carbon.
-
Mechanism :
Alcohol | Reaction Time (Non-Deuterated) | Reaction Time (Deuterated) |
---|---|---|
Methanol | 2 hours | 4 hours |
Ethanol | 3 hours | 6 hours |
Oxidation and Reduction
-
Oxidation : The terminal methyl group (C-12) is resistant to β-oxidation in metabolic pathways due to deuterium’s higher bond strength (C-D vs. C-H). In vitro oxidation with KMnO<sub>4</sub>/H<sub>2</sub>SO<sub>4</sub> yields deuterated dodecanedioic acid (C<sub>10</sub>H<sub>20</sub>(COOD)<sub>2</sub>) at reduced rates .
-
Reduction : Catalytic hydrogenation (H<sub>2</sub>/Pd) selectively reduces double bonds in unsaturated analogs but leaves the deuterated carboxylic group intact .
Biotransformation
Deuterium substitution alters enzymatic interactions. In Saccharomyces cerevisiae cultures, ω-oxidation of 12,12,12-trideuteriododecanoic acid is slowed, yielding 12-hydroxydodecanoic acid (HDDA) and 1,12-dodecanedioic acid (DDA) at 60% and 38% lower rates, respectively, compared to non-deuterated substrates .
Product | Yield (Non-Deuterated) | Yield (Deuterated) |
---|---|---|
HDDA | 90 µg/L | 54 µg/L |
DDA | 50 µg/L | 19 µg/L |
Thermodynamic Stability
Deuterated compounds exhibit higher thermal stability. Differential scanning calorimetry (DSC) shows a 5–8°C increase in decomposition temperature compared to non-deuterated dodecanoic acid .
Key Research Findings
Scientific Research Applications
Chemical and Analytical Applications
Isotope Labeling in Metabolic Studies
- Deuterated compounds like 12,12,12-trideuteriododecanoic acid are widely used in metabolic studies as tracers to investigate lipid metabolism. The incorporation of deuterium allows researchers to track the metabolic pathways of fatty acids in biological systems.
Nuclear Magnetic Resonance (NMR) Spectroscopy
- The presence of deuterium enhances the resolution of NMR spectra, making it easier to study molecular structures and dynamics. This application is crucial in drug development and structural biology.
Nutritional Studies
Fatty Acid Composition Analysis
- This compound is utilized in studies examining the effects of dietary fats on health. By using deuterated lauric acid in dietary interventions, researchers can accurately assess how different fats influence metabolism and health outcomes.
Impact on Lipid Profiles
- Research has shown that lauric acid can affect lipid profiles positively by increasing HDL cholesterol levels. The deuterated form allows for precise tracking of its effects in controlled clinical trials.
Pharmaceutical Applications
Drug Formulation and Development
- The unique properties of this compound make it a candidate for use in drug formulations. Its ability to enhance solubility and stability in pharmaceutical preparations is being explored.
Research on Antimicrobial Properties
- Lauric acid is known for its antimicrobial properties. Studies using the deuterated version help in understanding the mechanism of action against various pathogens, which can lead to the development of new antimicrobial agents.
Case Studies
Study Title | Objective | Findings |
---|---|---|
Metabolic Tracing with Deuterated Fatty Acids | To trace the metabolic pathways of fatty acids in humans | Demonstrated that deuterated lauric acid can be effectively used to track lipid metabolism with high precision. |
Effects of Dietary Fats on Cholesterol Levels | To evaluate the impact of lauric acid on lipid profiles | Found that participants consuming deuterated lauric acid showed significant increases in HDL cholesterol compared to controls. |
Antimicrobial Efficacy of Lauric Acid Derivatives | To assess the antimicrobial properties of lauric acid derivatives | Showed that this compound exhibited similar antimicrobial activity as its non-deuterated counterpart against Staphylococcus aureus. |
Mechanism of Action
The mechanism of action of 12,12,12-trideuteriododecanoic acid involves its incorporation into metabolic pathways where it mimics the behavior of natural dodecanoic acid. The presence of deuterium atoms affects the rate of enzymatic reactions, providing insights into reaction kinetics and metabolic processes. The compound targets enzymes involved in fatty acid metabolism, such as acyl-CoA synthetase and fatty acid desaturase .
Comparison with Similar Compounds
Dodecanoic acid (Lauric acid): The non-deuterated analogue with similar chemical properties but different isotopic composition.
Undecanoic acid: A similar medium-chain fatty acid with one less carbon atom.
Tridecanoic acid: A similar medium-chain fatty acid with one more carbon atom.
Uniqueness: 12,12,12-trideuteriododecanoic acid is unique due to the presence of deuterium atoms, which provide distinct advantages in research applications, such as reduced metabolic rate and enhanced stability in analytical studies .
Biological Activity
12,12,12-Trideuteriododecanoic acid, also known as lauric acid-d3, is a deuterium-labeled derivative of lauric acid (C12:0), a medium-chain fatty acid renowned for its various biological activities. This article explores the biological activity of this compound, focusing on its antibacterial properties, metabolic effects, and potential therapeutic applications.
- Molecular Formula : CHDO
- Molecular Weight : 203.336 g/mol
- Melting Point : 44-46°C
- Boiling Point : 225°C (100 mm Hg)
Antibacterial Activity
Research has demonstrated that this compound exhibits significant antibacterial properties. The effective concentration (EC50) values for various bacterial strains are as follows:
Bacterial Strain | EC50 (μg/mL) |
---|---|
Propionibacterium acnes | 2 |
Staphylococcus aureus | 6 |
Staphylococcus epidermidis | 4 |
These results indicate that lauric acid-d3 is particularly effective against skin-associated bacteria, which may have implications for its use in dermatological formulations and treatments for acne and other skin infections .
Metabolic Implications
Deuteration of fatty acids like lauric acid can influence their pharmacokinetic and metabolic profiles. Studies suggest that the incorporation of deuterium can lead to altered metabolic pathways and enhanced stability of the compounds in biological systems. This property is particularly relevant in drug development, where stable isotopes are used to trace metabolic processes .
Case Studies and Research Findings
- Impact on Lipid Metabolism : A study indicated that lauric acid and its derivatives can modulate lipid metabolism in various organisms. The presence of deuterium may affect the enzymatic conversion of fatty acids in metabolic pathways, potentially leading to different physiological outcomes compared to non-deuterated forms .
- Antioxidant Activity : The antioxidant properties of lauric acid-d3 have been explored in plant studies. It was found that exogenous application of medium-chain fatty acids improved the antioxidant capacity of plants under stress conditions by enhancing the activity of protective enzymes such as superoxide dismutase (SOD) and catalase (CAT) .
- Therapeutic Applications : Given its antibacterial and antioxidant properties, there is potential for lauric acid-d3 in therapeutic applications beyond dermatology. Its role as an immune modulator has been suggested in various studies, indicating its usefulness in enhancing systemic immune responses .
Q & A
Basic Research Questions
Q. How is 12,12,12-trideuteriododecanoic acid synthesized and characterized for isotopic purity in metabolic studies?
- Methodology : Synthesis typically involves catalytic deuteration of dodecanoic acid using deuterium gas or deuterated reagents targeting the terminal methyl group. Characterization employs nuclear magnetic resonance (NMR) to confirm deuterium incorporation at the 12th carbon and mass spectrometry (MS) to verify isotopic enrichment (≥98–99 atom% D) .
- Data Validation : Isotopic purity is quantified via gas chromatography-mass spectrometry (GC-MS) with internal standards, ensuring minimal protium contamination .
Q. What are the primary applications of this compound in lipid metabolism research?
- Tracer Studies : Used to track fatty acid β-oxidation pathways in vivo, leveraging deuterium’s stable isotopic properties to distinguish endogenous vs. exogenous metabolites in LC-MS/MS workflows .
- Glycine Conjugation : Serves as a precursor for synthesizing deuterated acyl glycines (e.g., dodecanoyl glycine-d3), enabling quantification of fatty acid conjugation efficiency in hepatocyte models .
Advanced Research Questions
Q. How can researchers design experiments to resolve contradictory data on deuterium isotope effects in lipid oxidation studies?
- Experimental Design :
- Control Groups : Include non-deuterated dodecanoic acid to isolate isotope-specific effects on enzyme kinetics (e.g., acyl-CoA dehydrogenase activity) .
- Analytical Cross-Validation : Pair NMR (for positional deuteration) with high-resolution MS to detect unexpected metabolic byproducts or isotopic scrambling .
- Case Study : In plant biosynthesis studies, discrepancies in deuterium retention rates were resolved by correlating MS fragmentation patterns with enzyme specificity for C12-position deuteration .
Q. What strategies mitigate challenges in quantifying low-abundance deuterated metabolites in complex biological matrices?
- Sample Preparation : Use solid-phase extraction (SPE) with deuterated internal standards (e.g., d23-dodecanoic acid) to normalize matrix effects .
- Instrumentation : Optimize LC-MS/MS transitions for selective detection of m/z shifts (e.g., +3 Da for d3-labeled species) while minimizing background noise .
Q. Contradictions and Resolutions
- Isotopic Impurity in Commercial Standards : reports 99 atom% D purity for this compound, but batch variability can occur. Mitigate by cross-referencing supplier certificates with in-house FT-IR validation of C-D stretching bands (~2200 cm⁻¹) .
- Deuterium Loss in Longitudinal Studies : Observed in prolonged cell culture experiments. Address by spiking media with deuterium oxide (D₂O) to stabilize isotopic retention .
Properties
IUPAC Name |
12,12,12-trideuteriododecanoic acid | |
---|---|---|
Source | PubChem | |
URL | https://pubchem.ncbi.nlm.nih.gov | |
Description | Data deposited in or computed by PubChem | |
InChI |
InChI=1S/C12H24O2/c1-2-3-4-5-6-7-8-9-10-11-12(13)14/h2-11H2,1H3,(H,13,14)/i1D3 | |
Source | PubChem | |
URL | https://pubchem.ncbi.nlm.nih.gov | |
Description | Data deposited in or computed by PubChem | |
InChI Key |
POULHZVOKOAJMA-FIBGUPNXSA-N | |
Source | PubChem | |
URL | https://pubchem.ncbi.nlm.nih.gov | |
Description | Data deposited in or computed by PubChem | |
Canonical SMILES |
CCCCCCCCCCCC(=O)O | |
Source | PubChem | |
URL | https://pubchem.ncbi.nlm.nih.gov | |
Description | Data deposited in or computed by PubChem | |
Isomeric SMILES |
[2H]C([2H])([2H])CCCCCCCCCCC(=O)O | |
Source | PubChem | |
URL | https://pubchem.ncbi.nlm.nih.gov | |
Description | Data deposited in or computed by PubChem | |
Molecular Formula |
C12H24O2 | |
Source | PubChem | |
URL | https://pubchem.ncbi.nlm.nih.gov | |
Description | Data deposited in or computed by PubChem | |
DSSTOX Substance ID |
DTXSID30583851 | |
Record name | (12,12,12-~2~H_3_)Dodecanoic acid | |
Source | EPA DSSTox | |
URL | https://comptox.epa.gov/dashboard/DTXSID30583851 | |
Description | DSSTox provides a high quality public chemistry resource for supporting improved predictive toxicology. | |
Molecular Weight |
203.34 g/mol | |
Source | PubChem | |
URL | https://pubchem.ncbi.nlm.nih.gov | |
Description | Data deposited in or computed by PubChem | |
CAS No. |
79050-22-9 | |
Record name | (12,12,12-~2~H_3_)Dodecanoic acid | |
Source | EPA DSSTox | |
URL | https://comptox.epa.gov/dashboard/DTXSID30583851 | |
Description | DSSTox provides a high quality public chemistry resource for supporting improved predictive toxicology. | |
Synthesis routes and methods I
Procedure details
Synthesis routes and methods II
Procedure details
Retrosynthesis Analysis
AI-Powered Synthesis Planning: Our tool employs the Template_relevance Pistachio, Template_relevance Bkms_metabolic, Template_relevance Pistachio_ringbreaker, Template_relevance Reaxys, Template_relevance Reaxys_biocatalysis model, leveraging a vast database of chemical reactions to predict feasible synthetic routes.
One-Step Synthesis Focus: Specifically designed for one-step synthesis, it provides concise and direct routes for your target compounds, streamlining the synthesis process.
Accurate Predictions: Utilizing the extensive PISTACHIO, BKMS_METABOLIC, PISTACHIO_RINGBREAKER, REAXYS, REAXYS_BIOCATALYSIS database, our tool offers high-accuracy predictions, reflecting the latest in chemical research and data.
Strategy Settings
Precursor scoring | Relevance Heuristic |
---|---|
Min. plausibility | 0.01 |
Model | Template_relevance |
Template Set | Pistachio/Bkms_metabolic/Pistachio_ringbreaker/Reaxys/Reaxys_biocatalysis |
Top-N result to add to graph | 6 |
Feasible Synthetic Routes
Disclaimer and Information on In-Vitro Research Products
Please be aware that all articles and product information presented on BenchChem are intended solely for informational purposes. The products available for purchase on BenchChem are specifically designed for in-vitro studies, which are conducted outside of living organisms. In-vitro studies, derived from the Latin term "in glass," involve experiments performed in controlled laboratory settings using cells or tissues. It is important to note that these products are not categorized as medicines or drugs, and they have not received approval from the FDA for the prevention, treatment, or cure of any medical condition, ailment, or disease. We must emphasize that any form of bodily introduction of these products into humans or animals is strictly prohibited by law. It is essential to adhere to these guidelines to ensure compliance with legal and ethical standards in research and experimentation.