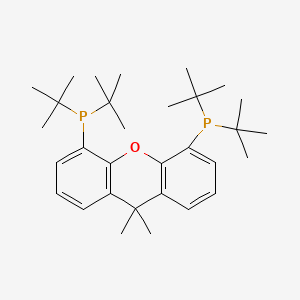
9,9-Dimethyl-4,5-bis(di-tert-butylphosphino)xanthene
Overview
Description
9,9-Dimethyl-4,5-bis(di-tert-butylphosphino)xanthene: is a phosphine ligand widely used in coordination chemistry and catalysis. It is known for its steric bulk and electron-donating properties, which make it an effective ligand in various metal-catalyzed reactions. The compound’s structure includes a xanthene backbone with two di-tert-butylphosphino groups attached at the 4 and 5 positions, and two methyl groups at the 9 position.
Mechanism of Action
Target of Action
The primary target of 9,9-Dimethyl-4,5-bis(di-tert-butylphosphino)xanthene, also known as t-Bu-Xantphos, is to act as a ligand in various metal-catalyzed reactions . It is used in the cobalt-catalyzed alkenylzincation of unfunctionalized alkynes and the cobalt-catalyzed alkylboration of alkenes .
Mode of Action
t-Bu-Xantphos interacts with its targets by binding to the metal catalysts used in the reactions. This interaction enhances the efficiency of the reactions and results in the formation of new compounds .
Biochemical Pathways
The affected pathways involve the catalytic cycles of the metal-catalyzed reactions. The presence of t-Bu-Xantphos influences these pathways by facilitating the formation of the desired products .
Pharmacokinetics
Its impact on bioavailability is significant in the context of these reactions, as it aids in the efficient conversion of reactants to products .
Result of Action
The molecular and cellular effects of t-Bu-Xantphos’s action are observed in the products of the reactions it catalyzes. For instance, it is used in the palladium-catalyzed N-alkylation of amines using primary and secondary alcohols, resulting in the formation of new alkylated amine compounds .
Biochemical Analysis
Biochemical Properties
9,9-Dimethyl-4,5-bis(di-tert-butylphosphino)xanthene plays a crucial role in biochemical reactions, primarily as a ligand in coordination chemistry. It interacts with various enzymes, proteins, and other biomolecules, forming stable complexes that facilitate catalytic processes. For instance, it is known to form complexes with copper and silver ions, which are used in photophysical studies . These interactions are typically characterized by the formation of coordination bonds between the phosphine groups of this compound and the metal ions, leading to enhanced stability and reactivity of the resulting complexes.
Cellular Effects
The effects of this compound on various types of cells and cellular processes are significant. It influences cell function by modulating cell signaling pathways, gene expression, and cellular metabolism. Studies have shown that its complexes can affect the photophysical properties of cells, potentially altering their behavior under specific conditions . Additionally, the presence of this compound in cellular environments can lead to changes in the expression of genes involved in metabolic pathways, thereby impacting overall cellular function.
Molecular Mechanism
At the molecular level, this compound exerts its effects through binding interactions with biomolecules. It acts as a ligand, forming coordination complexes with metal ions, which can either inhibit or activate specific enzymes. These interactions often result in changes in gene expression, as the complexes can influence transcription factors and other regulatory proteins. The binding of this compound to metal ions also enhances the stability and reactivity of the complexes, facilitating various biochemical reactions .
Temporal Effects in Laboratory Settings
In laboratory settings, the effects of this compound change over time. The stability of the compound is generally high, but it can degrade under certain conditions, such as exposure to light or extreme temperatures . Long-term studies have shown that its complexes can have lasting effects on cellular function, with some changes in gene expression and metabolic activity persisting even after the compound is no longer present. These temporal effects are crucial for understanding the long-term implications of using this compound in biochemical research.
Dosage Effects in Animal Models
The effects of this compound vary with different dosages in animal models. At low doses, the compound can enhance certain biochemical reactions without causing significant adverse effects. At higher doses, it may exhibit toxic effects, including disruptions in cellular metabolism and gene expression . Threshold effects have been observed, where a specific dosage level leads to a marked change in the compound’s impact on cellular function. Understanding these dosage effects is essential for optimizing the use of this compound in research and therapeutic applications.
Metabolic Pathways
This compound is involved in various metabolic pathways, interacting with enzymes and cofactors that regulate metabolic flux and metabolite levels. Its complexes with metal ions can influence the activity of enzymes involved in key metabolic processes, such as glycolysis and the citric acid cycle . These interactions can lead to changes in the levels of metabolites, affecting overall cellular metabolism. The role of this compound in these pathways highlights its importance in biochemical research.
Transport and Distribution
Within cells and tissues, this compound is transported and distributed through interactions with transporters and binding proteins. These interactions facilitate its localization to specific cellular compartments, where it can exert its effects . The distribution of this compound within cells is crucial for its function, as it needs to reach target sites to form effective complexes with metal ions and other biomolecules.
Subcellular Localization
The subcellular localization of this compound is influenced by targeting signals and post-translational modifications that direct it to specific compartments or organelles. These localization patterns can affect the compound’s activity and function, as it needs to be in the right place to interact with its target biomolecules . Understanding the subcellular distribution of this compound is essential for elucidating its role in biochemical processes.
Preparation Methods
Synthetic Routes and Reaction Conditions: The synthesis of 9,9-Dimethyl-4,5-bis(di-tert-butylphosphino)xanthene typically involves the reaction of 9,9-dimethylxanthene with di-tert-butylphosphine. The reaction is usually carried out in the presence of a base such as sodium hydride or potassium tert-butoxide to deprotonate the phosphine and facilitate the formation of the phosphine ligand.
Industrial Production Methods: While specific industrial production methods are not widely documented, the synthesis generally follows similar principles as laboratory-scale preparation, with adjustments for larger scale and efficiency. The use of continuous flow reactors and optimized reaction conditions can enhance the yield and purity of the compound.
Chemical Reactions Analysis
Types of Reactions: 9,9-Dimethyl-4,5-bis(di-tert-butylphosphino)xanthene undergoes various types of reactions, including:
Oxidation: The phosphine groups can be oxidized to phosphine oxides.
Substitution: The compound can participate in substitution reactions where the phosphine ligands are replaced by other ligands.
Common Reagents and Conditions:
Oxidation: Common oxidizing agents include hydrogen peroxide and oxygen.
Substitution: Typical reagents include halides and other nucleophiles.
Major Products:
Oxidation: The major product is the corresponding phosphine oxide.
Substitution: The products depend on the nature of the substituting ligand.
Scientific Research Applications
Chemistry: 9,9-Dimethyl-4,5-bis(di-tert-butylphosphino)xanthene is used as a ligand in various metal-catalyzed reactions, including cross-coupling reactions such as Suzuki-Miyaura, Heck, and Stille couplings . It is also employed in hydroformylation and hydrogenation reactions.
Biology and Medicine: While its direct applications in biology and medicine are limited, the compound’s role in catalysis can indirectly contribute to the synthesis of biologically active molecules and pharmaceuticals.
Industry: In the industrial sector, the compound is used in the production of fine chemicals and materials. Its ability to stabilize metal complexes makes it valuable in processes requiring precise control over reaction conditions and product selectivity.
Comparison with Similar Compounds
Xantphos: A related ligand with a similar xanthene backbone but different substituents.
tBuXPhos: Another phosphine ligand with tert-butyl groups but different structural features.
RuPhos: A phosphine ligand used in similar catalytic applications.
Uniqueness: 9,9-Dimethyl-4,5-bis(di-tert-butylphosphino)xanthene is unique due to its specific combination of steric bulk and electron-donating properties, which provide distinct advantages in certain catalytic reactions. Its ability to form stable complexes with a variety of metals makes it versatile and effective in numerous applications.
Properties
IUPAC Name |
ditert-butyl-(5-ditert-butylphosphanyl-9,9-dimethylxanthen-4-yl)phosphane | |
---|---|---|
Source | PubChem | |
URL | https://pubchem.ncbi.nlm.nih.gov | |
Description | Data deposited in or computed by PubChem | |
InChI |
InChI=1S/C31H48OP2/c1-27(2,3)33(28(4,5)6)23-19-15-17-21-25(23)32-26-22(31(21,13)14)18-16-20-24(26)34(29(7,8)9)30(10,11)12/h15-20H,1-14H3 | |
Source | PubChem | |
URL | https://pubchem.ncbi.nlm.nih.gov | |
Description | Data deposited in or computed by PubChem | |
InChI Key |
ZEIZANJFJXHMNS-UHFFFAOYSA-N | |
Source | PubChem | |
URL | https://pubchem.ncbi.nlm.nih.gov | |
Description | Data deposited in or computed by PubChem | |
Canonical SMILES |
CC1(C2=C(C(=CC=C2)P(C(C)(C)C)C(C)(C)C)OC3=C1C=CC=C3P(C(C)(C)C)C(C)(C)C)C | |
Source | PubChem | |
URL | https://pubchem.ncbi.nlm.nih.gov | |
Description | Data deposited in or computed by PubChem | |
Molecular Formula |
C31H48OP2 | |
Source | PubChem | |
URL | https://pubchem.ncbi.nlm.nih.gov | |
Description | Data deposited in or computed by PubChem | |
DSSTOX Substance ID |
DTXSID50584820 | |
Record name | (9,9-Dimethyl-9H-xanthene-4,5-diyl)bis(di-tert-butylphosphane) | |
Source | EPA DSSTox | |
URL | https://comptox.epa.gov/dashboard/DTXSID50584820 | |
Description | DSSTox provides a high quality public chemistry resource for supporting improved predictive toxicology. | |
Molecular Weight |
498.7 g/mol | |
Source | PubChem | |
URL | https://pubchem.ncbi.nlm.nih.gov | |
Description | Data deposited in or computed by PubChem | |
CAS No. |
856405-77-1 | |
Record name | (9,9-Dimethyl-9H-xanthene-4,5-diyl)bis(di-tert-butylphosphane) | |
Source | EPA DSSTox | |
URL | https://comptox.epa.gov/dashboard/DTXSID50584820 | |
Description | DSSTox provides a high quality public chemistry resource for supporting improved predictive toxicology. | |
Retrosynthesis Analysis
AI-Powered Synthesis Planning: Our tool employs the Template_relevance Pistachio, Template_relevance Bkms_metabolic, Template_relevance Pistachio_ringbreaker, Template_relevance Reaxys, Template_relevance Reaxys_biocatalysis model, leveraging a vast database of chemical reactions to predict feasible synthetic routes.
One-Step Synthesis Focus: Specifically designed for one-step synthesis, it provides concise and direct routes for your target compounds, streamlining the synthesis process.
Accurate Predictions: Utilizing the extensive PISTACHIO, BKMS_METABOLIC, PISTACHIO_RINGBREAKER, REAXYS, REAXYS_BIOCATALYSIS database, our tool offers high-accuracy predictions, reflecting the latest in chemical research and data.
Strategy Settings
Precursor scoring | Relevance Heuristic |
---|---|
Min. plausibility | 0.01 |
Model | Template_relevance |
Template Set | Pistachio/Bkms_metabolic/Pistachio_ringbreaker/Reaxys/Reaxys_biocatalysis |
Top-N result to add to graph | 6 |
Feasible Synthetic Routes
Q1: What makes t-Bu-Xantphos unique compared to other diphosphine ligands in transition metal catalysis?
A: t-Bu-Xantphos distinguishes itself with its bulky tert-butyl substituents and rigid xanthene backbone. [] These structural features translate into a larger natural bite angle compared to similar ligands like Ph-Xantphos. [] This larger bite angle influences the reactivity and selectivity of the metal complexes it forms, often leading to enhanced catalytic activity. [, ] For example, in platinum-catalyzed direct amination reactions, t-Bu-Xantphos, despite its large bite angle, showed lower activity compared to Xantphos, highlighting that other factors like the substituents on the phosphorous atoms also play a crucial role. []
Q2: How does the oxygen atom in the xanthene backbone of t-Bu-Xantphos contribute to its coordination chemistry?
A: The oxygen atom can act as an additional donor to the metal center, leading to the formation of unique pincer complexes. [] This hemilabile behavior is observed with metals like rhodium and platinum. [] The trans influence of the oxygen donor atom was found to be dependent on the bridgehead atom in the xanthene backbone, with t-Bu-sixantphos < t-Bu-thixantphos < t-Bu-xantphos. []
Q3: Can you provide examples of reactions where t-Bu-Xantphos-metal complexes have demonstrated catalytic activity?
A: t-Bu-Xantphos has found applications in various catalytic transformations. For instance, a copper(I) alkoxide complex with t-Bu-Xantphos effectively catalyzes the alkynylation of trifluoromethyl ketones. [] Another example is the copper(I)-catalyzed intramolecular hydroamination of alkenes, where t-Bu-Xantphos-based catalysts facilitate the formation of pyrrolidine and piperidine derivatives with high efficiency. [] Additionally, a gold(I) chloride complex, tBuXantphosAuCl, showed promising activity in the catalytic transformation of the C-F bond of aromatic substrates to C-X (X = O, S, N) bonds. []
Q4: What is the significance of the longer Au-Cl bond length observed in the tBuXantphosAuCl complex?
A: The significantly longer Au-Cl bond in tBuXantphosAuCl compared to other gold complexes suggests a weaker bond. [] This weakening is attributed to the steric bulk of the t-Bu-Xantphos ligand and its influence on the coordination geometry around the gold center. [] This weaker Au-Cl bond potentially facilitates ligand exchange reactions, a crucial aspect of its catalytic activity. []
Q5: Have there been any investigations into the structural rigidity of t-Bu-Xantphos complexes under high pressure?
A: Single crystal X-ray diffraction studies on copper(I) and silver(I) complexes of t-Bu-Xantphos under ambient and elevated pressure conditions showed minimal structural changes with increasing pressure. [] This finding suggests a high degree of structural rigidity, a desirable property for potential applications in high-pressure catalytic processes.
Q6: Has t-Bu-Xantphos been explored in the context of halogen bonding interactions?
A: Interestingly, a study on a trans-dibromogold(III) t-Bu-Xantphos complex revealed the presence of attractive Br···Br interactions in the solid state. [] These interactions drive the self-assembly of the complex into infinite 1-dimensional chains. [] DFT calculations and topological analysis of the electron density further support the presence and significance of these halogen bonding interactions. []
Disclaimer and Information on In-Vitro Research Products
Please be aware that all articles and product information presented on BenchChem are intended solely for informational purposes. The products available for purchase on BenchChem are specifically designed for in-vitro studies, which are conducted outside of living organisms. In-vitro studies, derived from the Latin term "in glass," involve experiments performed in controlled laboratory settings using cells or tissues. It is important to note that these products are not categorized as medicines or drugs, and they have not received approval from the FDA for the prevention, treatment, or cure of any medical condition, ailment, or disease. We must emphasize that any form of bodily introduction of these products into humans or animals is strictly prohibited by law. It is essential to adhere to these guidelines to ensure compliance with legal and ethical standards in research and experimentation.