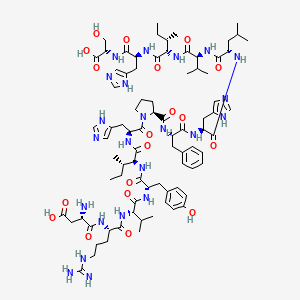
Serine human angiotensin tetradecapeptide
Overview
Description
Serine human angiotensin tetradecapeptide, also known as Renin Substrate Tetradecapeptide (Human) or Angiotensinogen-(1–14), is a 14-amino acid peptide (Asp-Arg-Val-Tyr-Ile-His-Pro-Phe-His-Leu-Val-Ile-His-Asn) with a molecular weight of 1758.94 Da . It serves as the primary substrate for renin in the renin-angiotensin system (RAS), a critical pathway regulating blood pressure and fluid balance. Upon cleavage by renin between residues Leu¹⁰ and Val¹¹, it generates angiotensin I (Ang I, a decapeptide), which is further processed by angiotensin-converting enzyme (ACE) into angiotensin II (Ang II), a potent vasoconstrictor .
This peptide is widely used in biochemical assays to study renin activity and screen renin inhibitors, employing techniques such as high-performance liquid chromatography (HPLC) and mass spectrometry (MS) . Its standardized sequence and commercial availability (e.g., Phoenix Biotech Catalog #002-45) make it a cornerstone in cardiovascular research .
Preparation Methods
Synthetic Routes and Reaction Conditions: The synthesis of serine human angiotensin tetradecapeptide typically involves solid-phase peptide synthesis (SPPS). This method allows for the sequential addition of amino acids to a growing peptide chain anchored to a solid resin. The process includes:
Activation of Amino Acids: Using reagents like dicyclohexylcarbodiimide (DCC) or N,N’-diisopropylcarbodiimide (DIC) to activate the carboxyl group of the incoming amino acid.
Coupling Reaction: The activated amino acid is coupled to the resin-bound peptide chain.
Deprotection: Removal of protecting groups from the amino acids to allow for subsequent coupling reactions.
Industrial Production Methods: Industrial production of this compound may involve large-scale SPPS or recombinant DNA technology. In recombinant DNA technology, the gene encoding the peptide is inserted into a suitable expression system, such as Escherichia coli or yeast, which then produces the peptide in large quantities. The peptide is subsequently purified using chromatographic techniques .
Types of Reactions:
Oxidation: this compound can undergo oxidation, particularly at methionine residues, leading to the formation of methionine sulfoxide.
Reduction: Reduction reactions can reverse oxidation, restoring methionine residues to their original state.
Substitution: Amino acid residues within the peptide can be substituted to create analogs with different biological activities.
Common Reagents and Conditions:
Oxidizing Agents: Hydrogen peroxide or performic acid for oxidation reactions.
Reducing Agents: Dithiothreitol (DTT) or tris(2-carboxyethyl)phosphine (TCEP) for reduction reactions.
Substitution Reagents: Amino acid derivatives and coupling reagents like DCC or DIC for substitution reactions.
Major Products:
Oxidation Products: Methionine sulfoxide-containing peptides.
Reduction Products: Restored methionine residues.
Substitution Products: Peptide analogs with modified amino acid sequences.
Scientific Research Applications
Serine human angiotensin tetradecapeptide has a wide range of scientific research applications:
Chemistry: Used as a model peptide for studying peptide synthesis and modification techniques.
Biology: Investigated for its role in the renin-angiotensin system and its effects on blood pressure regulation.
Medicine: Explored for potential therapeutic applications in treating hypertension and cardiovascular diseases.
Mechanism of Action
The mechanism of action of serine human angiotensin tetradecapeptide involves its interaction with angiotensin receptors, primarily angiotensin II type 1 (AT1) receptors. Upon binding to these receptors, the peptide triggers a cascade of intracellular signaling pathways that lead to vasoconstriction, increased blood pressure, and aldosterone secretion. These effects are mediated through the activation of G-protein-coupled receptors and subsequent activation of phospholipase C, which increases intracellular calcium levels .
Comparison with Similar Compounds
Structural Analogues: Species-Specific Renin Substrate Tetradecapeptides
The tetradecapeptide exists in species-specific variants (e.g., human, horse, rat), with sequence variations influencing renin specificity. For example:
- Human : Asp-Arg-Val-Tyr-Ile-His-Pro-Phe-His-Leu-Val-Ile-His-Asn .
- Rat : Substitutions in residues critical for renin binding (e.g., His⁶, Leu¹⁰) may alter cleavage kinetics .
Renin isoforms (I–IV) exhibit similar Michaelis constants (Km = 0.71–1.76 ×10⁻⁶ M) for human tetradecapeptide, suggesting conserved substrate recognition across enzyme forms .
Functional Analogues: SFTI-1 Cyclic Tetradecapeptide
Sunflower Trypsin Inhibitor-1 (SFTI-1) is a disulfide-bridged cyclic tetradecapeptide with contrasting roles:
SFTI-1’s cyclic structure enhances protease resistance, whereas the linear tetradecapeptide’s flexibility facilitates renin-mediated cleavage .
Substrate Variants with Amino Acid Substitutions
Substitutions in the P2 position (e.g., Ser or Asp replacing Leu) drastically reduce cleavage efficiency by serine proteases:
P2 Position Residue | Cleavage Efficiency (Relative to Leu) | Enzyme Compatibility | Reference |
---|---|---|---|
Leu | 1× (Baseline) | High (Renin, Tonin) | |
Ser | 160× lower | Poor | |
Asp | Not cleaved | Incompatible |
These findings highlight the necessity of hydrophobic residues (e.g., Leu) in the P2 position for efficient proteolysis .
Related Peptides in the Angiotensin System
Angiotensin-(1–14) demonstrates higher conversion efficiency to Ang-(1–12) in rat aorta compared to Ang I production, suggesting tissue-specific processing pathways .
Other Tetradecapeptides with Diverse Functions
- Somatostatin: A 14-amino acid hormone (sequence: AGCKNFFWKTFTSC) inhibiting growth hormone release. Unlike the angiotensin tetradecapeptide, it is derived from a larger precursor (pre-prosomatostatin) and regulates endocrine functions .
- Phosphorylated Tetradecapeptides : Used in kinase/phosphatase assays (e.g., [³²P]-labeled variants) to study enzymatic inhibition (e.g., EDTA reduces phosphatase activity by 50% at 25 mM) .
Research and Clinical Implications
- Drug Development : The tetradecapeptide is critical for screening renin inhibitors (e.g., SC-52310, Ki = 0.8 nM) .
- Diagnostics: Used in MS-based assays for RAS component quantification .
- Therapeutic Targets : Modulating angiotensin processing enzymes (e.g., chymase) could offer alternatives to ACE inhibitors .
Biological Activity
Serine human angiotensin tetradecapeptide (Angiotensin I, or Ang I) is a crucial component of the renin-angiotensin system (RAS), which plays a significant role in regulating blood pressure and fluid balance in the human body. This peptide, consisting of 14 amino acids, is generated from angiotensinogen by the action of renin and subsequently converted to angiotensin II (Ang II) by angiotensin-converting enzyme (ACE). The biological activity of Ang I and its derivatives is essential for understanding various cardiovascular diseases, including hypertension.
The structure of Ang I is pivotal for its interaction with the RAS. It serves as a substrate for ACE, which cleaves it to produce Ang II, a potent vasoconstrictor. The binding affinity and specificity of Ang I towards ACE are influenced by its amino acid sequence and conformation. Recent studies have elucidated the molecular interactions involved in this process, revealing that modifications to the peptide can significantly alter its biological activity.
Table 1: Comparison of Angiotensin Peptides
Peptide | Sequence | Function |
---|---|---|
Angiotensin I | Asp-Arg-Val-Tyr-Ile-His-Pro-Phe-Thr-Ser-Leu | Precursor to Ang II |
Angiotensin II | Asp-Arg-Val-Tyr-Ile-His-Pro-Phe | Vasoconstriction and blood pressure regulation |
Angiotensin III | Arg-Val-Tyr-Ile-His-Pro-Phe | Similar to Ang II but with lower potency |
Biological Activity
The biological activity of this compound is primarily mediated through its conversion to Ang II, which binds to AT1 and AT2 receptors, leading to various physiological effects:
- Vasoconstriction : Ang II causes constriction of blood vessels, increasing blood pressure.
- Aldosterone Secretion : It stimulates adrenal glands to secrete aldosterone, promoting sodium retention and further increasing blood pressure.
- Sympathetic Nervous System Activation : Enhances sympathetic outflow, contributing to increased heart rate and contractility.
Case Studies
Several studies have investigated the implications of altered Ang I levels in clinical conditions:
- Hypertension : A study demonstrated that elevated plasma levels of angiotensinogen (precursor to Ang I) correlate with hypertension in patients with M235T polymorphism in the AGT gene. This polymorphism results in increased angiotensinogen production, leading to higher Ang I levels and subsequent hypertension .
- Heart Failure : Research indicates that patients with heart failure exhibit dysregulation in the RAS, characterized by elevated levels of both Ang I and Ang II. This dysregulation contributes to fluid overload and worsening cardiac function .
- Diabetes : In diabetic patients, increased renin activity has been observed, leading to higher production of Ang I. This can exacerbate hypertension and cardiovascular complications commonly seen in diabetes .
Research Findings
Recent advancements in understanding the biochemical pathways involving this compound have been significant:
- Inhibitors : Development of renin inhibitors has shown promise in treating hypertension by directly affecting the conversion of angiotensinogen to Ang I. These inhibitors can reduce blood pressure effectively without some side effects associated with ACE inhibitors .
- Fluorogenic Substrates : Innovative assays using fluorogenic substrates derived from the N-terminal sequence of human angiotensinogen have been developed for measuring renin activity with high sensitivity. This allows for better characterization of inhibitors and understanding their mechanisms .
Chemical Reactions Analysis
Structural Characteristics
Molecular formula : C₈₂H₁₂₁N₂₃O₁₉
Molecular weight : 1,733.0 g/mol
Sequence : DRVYIHPFHLVIHS
Key Structural Features:
-
N-terminal domain : Contains the angiotensin I (Ang I) sequence (Asp¹–His¹⁰).
-
C-terminal extension : Unique His¹¹–Ser¹⁴ residues influence substrate-enzyme interactions .
-
Disulfide bonds : Absent, differentiating it from full-length angiotensinogen .
Enzymatic Cleavage Reactions
SHAT undergoes proteolytic processing by multiple enzymes to generate bioactive angiotensin peptides:
Reaction Notes:
-
Renin specificity : Preferentially cleaves the Leu¹⁰–Val¹¹ bond but shows reduced activity on SHAT compared to full-length angiotensinogen (Km = 47.5 μM vs. 2.6 μM) .
-
Chymase dominance : Accounts for >80% of Ang II production in human cardiovascular tissues .
-
pH dependence : Cathepsin D exhibits maximal activity at pH 4.7, with reduced efficacy at physiological pH .
Kinetic Parameters
Data from competitive inhibition assays and substrate turnover studies:
Parameter | Renin | Cathepsin D | Chymase |
---|---|---|---|
Km (μM) | 47.5 | 12.3 | 8.9 |
Vmax (nmol/min) | 0.32 | 1.45 | 2.10 |
IC₅₀ (inhibitors) | 1.2 nM (H-77) | >10 μM (Pepstatin) | 0.8 nM (Chymostatin) |
Functional Implications
-
Hypertension models : SHAT-derived Ang II production persists in nephrectomized rats, indicating non-renal enzymatic pathways .
-
Species specificity : Human SHAT is poorly cleaved by rodent renin, necessitating transgenic models for cross-species studies .
-
Therapeutic targeting : Renin inhibitors (e.g., H-77) show 1,000-fold selectivity over cathepsin D in SHAT processing assays .
Analytical Detection Methods
-
Chromatography : Reverse-phase HPLC with UV detection (λ = 214 nm) .
-
Mass spectrometry : MALDI-TOF confirms cleavage products (Ang I: m/z 1,296.5; Ang II: m/z 1,046.2) .
This synthesis integrates structural, enzymatic, and functional data to elucidate SHAT's role in angiotensin peptide dynamics. Experimental discrepancies in catalytic rates highlight the importance of contextual factors like pH, redox state, and tissue-specific enzyme expression.
Q & A
Basic Research Questions
Q. What is the structural and functional role of serine human angiotensin tetradecapeptide in the renin-angiotensin system (RAS)?
- Methodological Answer : The tetradecapeptide serves as a precursor for angiotensin II synthesis. Its structure includes a serine residue at the N-terminal, critical for enzymatic cleavage by renin. To confirm its role, use in vitro enzymatic assays (e.g., HPLC-coupled mass spectrometry) to track cleavage products and quantify angiotensin I/II generation . Comparative studies with mutated peptides can isolate structural determinants of enzyme specificity .
Q. What standardized assays are recommended for quantifying this compound in biological samples?
- Methodological Answer : ELISA kits optimized for RAS peptides (e.g., human angiotensin I/II ELISA) are preferred for specificity. Validate assays via spike-recovery experiments in plasma/serum matrices to account for protease interference. Include controls for cross-reactivity with homologous peptides (e.g., angiotensin 1-9) . For kinetic studies, combine radioimmunoassay (RIA) with liquid chromatography for improved sensitivity .
Q. How do researchers isolate and purify this compound from tissue homogenates?
- Methodological Answer : Use acid-ethanol extraction to precipitate proteins, followed by solid-phase extraction (SPE) with C18 columns. Confirm purity via reverse-phase HPLC and MALDI-TOF mass spectrometry. For tissue-specific isoforms, employ immunoaffinity chromatography with monoclonal antibodies targeting the serine residue .
Advanced Research Questions
Q. How can experimental design address contradictions in reported enzymatic processing rates of this compound across studies?
- Methodological Answer : Discrepancies often arise from differences in pH, ionic strength, or co-factors (e.g., chloride ions). Replicate assays under standardized conditions (e.g., 37°C, pH 7.4) and include kinetic parameters (Km, Vmax) for renin and angiotensin-converting enzyme (ACE). Use statistical meta-analysis to reconcile data from heterogeneous studies, weighting results by sample size and methodological rigor .
Q. What strategies resolve conflicting data on the peptide’s vasoconstrictive effects in different vascular beds?
- Methodological Answer : Conduct comparative ex vivo studies using wire myography in isolated rat femoral arteries versus mesenteric beds. Control for endothelial modulation (e.g., nitric oxide synthase inhibition) and measure calcium flux via fluorescent dyes (e.g., Fura-2). Cross-validate with in vivo blood pressure telemetry in hypertensive models to contextualize tissue-specific responses .
Q. How can novel molecular dynamics (MD) simulations improve understanding of the peptide’s interaction with renin?
- Methodological Answer : Perform all-atom MD simulations using software like GROMACS, focusing on the serine residue’s hydrogen bonding with renin’s catalytic aspartate. Compare binding free energies (ΔG) between wild-type and mutant peptides via MM-PBSA calculations. Validate predictions with surface plasmon resonance (SPR) to measure real-time binding kinetics .
Q. What advanced techniques characterize post-translational modifications (PTMs) of this compound in pathological states?
- Methodological Answer : Use tandem mass spectrometry (LC-MS/MS) with electron-transfer dissociation (ETD) to identify PTMs like phosphorylation or oxidation. Pair with immunohistochemistry in tissue microarrays to localize modified peptides in disease models (e.g., renal fibrosis). Integrate multi-omics datasets (proteomics, transcriptomics) to link PTMs to RAS dysregulation .
Q. Methodological and Data Analysis Challenges
Q. How should researchers design controls to distinguish angiotensin tetradecapeptide-derived signaling from off-target ACE pathways?
- Methodological Answer : Use ACE inhibitors (e.g., captopril) and renin-specific antagonists (e.g., aliskiren) in parallel experiments. Measure downstream markers (e.g., aldosterone, cAMP) via ELISA or Western blot. Include siRNA knockdown of ACE or angiotensin receptors to isolate pathway-specific effects .
Q. What statistical approaches are optimal for analyzing dose-response variability in peptide-induced vascular reactivity?
- Methodological Answer : Apply nonlinear regression models (e.g., sigmoidal dose-response curves) to calculate EC50 values. Use mixed-effects models to account for inter-subject variability in animal/human studies. For small sample sizes, employ bootstrapping to estimate confidence intervals .
Q. How can systems biology models integrate disparate data on the peptide’s role in hypertension and renal disease?
- Methodological Answer : Develop kinetic models using software like COPASI, incorporating parameters for peptide synthesis, degradation, and receptor binding. Validate against longitudinal clinical data (e.g., blood pressure, glomerular filtration rate). Use sensitivity analysis to identify dominant pathways in disease progression .
Properties
IUPAC Name |
(3S)-3-amino-4-[[(2S)-5-carbamimidamido-1-[[(2S)-1-[[(2S)-1-[[(2S,3S)-1-[[(2S)-1-[(2S)-2-[[(2S)-1-[[(2S)-1-[[(2S)-1-[[(2S)-1-[[(2S,3S)-1-[[(2S)-1-[[(1S)-1-carboxy-2-hydroxyethyl]amino]-3-(1H-imidazol-5-yl)-1-oxopropan-2-yl]amino]-3-methyl-1-oxopentan-2-yl]amino]-3-methyl-1-oxobutan-2-yl]amino]-4-methyl-1-oxopentan-2-yl]amino]-3-(1H-imidazol-5-yl)-1-oxopropan-2-yl]amino]-1-oxo-3-phenylpropan-2-yl]carbamoyl]pyrrolidin-1-yl]-3-(1H-imidazol-5-yl)-1-oxopropan-2-yl]amino]-3-methyl-1-oxopentan-2-yl]amino]-3-(4-hydroxyphenyl)-1-oxopropan-2-yl]amino]-3-methyl-1-oxobutan-2-yl]amino]-1-oxopentan-2-yl]amino]-4-oxobutanoic acid | |
---|---|---|
Source | PubChem | |
URL | https://pubchem.ncbi.nlm.nih.gov | |
Description | Data deposited in or computed by PubChem | |
InChI |
InChI=1S/C82H121N23O19/c1-11-45(9)66(103-74(116)57(30-48-22-24-52(107)25-23-48)97-76(118)64(43(5)6)101-69(111)54(20-16-26-89-82(84)85)93-68(110)53(83)34-63(108)109)79(121)99-60(33-51-37-88-41-92-51)80(122)105-27-17-21-62(105)75(117)96-56(29-47-18-14-13-15-19-47)70(112)95-58(31-49-35-86-39-90-49)71(113)94-55(28-42(3)4)73(115)102-65(44(7)8)77(119)104-67(46(10)12-2)78(120)98-59(32-50-36-87-40-91-50)72(114)100-61(38-106)81(123)124/h13-15,18-19,22-25,35-37,39-46,53-62,64-67,106-107H,11-12,16-17,20-21,26-34,38,83H2,1-10H3,(H,86,90)(H,87,91)(H,88,92)(H,93,110)(H,94,113)(H,95,112)(H,96,117)(H,97,118)(H,98,120)(H,99,121)(H,100,114)(H,101,111)(H,102,115)(H,103,116)(H,104,119)(H,108,109)(H,123,124)(H4,84,85,89)/t45-,46-,53-,54-,55-,56-,57-,58-,59-,60-,61-,62-,64-,65-,66-,67-/m0/s1 | |
Source | PubChem | |
URL | https://pubchem.ncbi.nlm.nih.gov | |
Description | Data deposited in or computed by PubChem | |
InChI Key |
CQGAGLNDCXHGEL-SDDIQEGKSA-N | |
Source | PubChem | |
URL | https://pubchem.ncbi.nlm.nih.gov | |
Description | Data deposited in or computed by PubChem | |
Canonical SMILES |
CCC(C)C(C(=O)NC(CC1=CN=CN1)C(=O)N2CCCC2C(=O)NC(CC3=CC=CC=C3)C(=O)NC(CC4=CN=CN4)C(=O)NC(CC(C)C)C(=O)NC(C(C)C)C(=O)NC(C(C)CC)C(=O)NC(CC5=CN=CN5)C(=O)NC(CO)C(=O)O)NC(=O)C(CC6=CC=C(C=C6)O)NC(=O)C(C(C)C)NC(=O)C(CCCNC(=N)N)NC(=O)C(CC(=O)O)N | |
Source | PubChem | |
URL | https://pubchem.ncbi.nlm.nih.gov | |
Description | Data deposited in or computed by PubChem | |
Isomeric SMILES |
CC[C@H](C)[C@@H](C(=O)N[C@@H](CC1=CN=CN1)C(=O)N2CCC[C@H]2C(=O)N[C@@H](CC3=CC=CC=C3)C(=O)N[C@@H](CC4=CN=CN4)C(=O)N[C@@H](CC(C)C)C(=O)N[C@@H](C(C)C)C(=O)N[C@@H]([C@@H](C)CC)C(=O)N[C@@H](CC5=CN=CN5)C(=O)N[C@@H](CO)C(=O)O)NC(=O)[C@H](CC6=CC=C(C=C6)O)NC(=O)[C@H](C(C)C)NC(=O)[C@H](CCCNC(=N)N)NC(=O)[C@H](CC(=O)O)N | |
Source | PubChem | |
URL | https://pubchem.ncbi.nlm.nih.gov | |
Description | Data deposited in or computed by PubChem | |
Molecular Formula |
C82H121N23O19 | |
Source | PubChem | |
URL | https://pubchem.ncbi.nlm.nih.gov | |
Description | Data deposited in or computed by PubChem | |
DSSTOX Substance ID |
DTXSID70238813 | |
Record name | Serine human angiotensin tetradecapeptide | |
Source | EPA DSSTox | |
URL | https://comptox.epa.gov/dashboard/DTXSID70238813 | |
Description | DSSTox provides a high quality public chemistry resource for supporting improved predictive toxicology. | |
Molecular Weight |
1733.0 g/mol | |
Source | PubChem | |
URL | https://pubchem.ncbi.nlm.nih.gov | |
Description | Data deposited in or computed by PubChem | |
CAS No. |
91999-74-5 | |
Record name | Serine human angiotensin tetradecapeptide | |
Source | ChemIDplus | |
URL | https://pubchem.ncbi.nlm.nih.gov/substance/?source=chemidplus&sourceid=0091999745 | |
Description | ChemIDplus is a free, web search system that provides access to the structure and nomenclature authority files used for the identification of chemical substances cited in National Library of Medicine (NLM) databases, including the TOXNET system. | |
Record name | Serine human angiotensin tetradecapeptide | |
Source | EPA DSSTox | |
URL | https://comptox.epa.gov/dashboard/DTXSID70238813 | |
Description | DSSTox provides a high quality public chemistry resource for supporting improved predictive toxicology. | |
Disclaimer and Information on In-Vitro Research Products
Please be aware that all articles and product information presented on BenchChem are intended solely for informational purposes. The products available for purchase on BenchChem are specifically designed for in-vitro studies, which are conducted outside of living organisms. In-vitro studies, derived from the Latin term "in glass," involve experiments performed in controlled laboratory settings using cells or tissues. It is important to note that these products are not categorized as medicines or drugs, and they have not received approval from the FDA for the prevention, treatment, or cure of any medical condition, ailment, or disease. We must emphasize that any form of bodily introduction of these products into humans or animals is strictly prohibited by law. It is essential to adhere to these guidelines to ensure compliance with legal and ethical standards in research and experimentation.