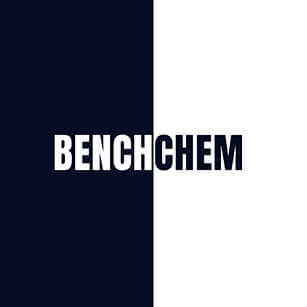
Acetonitrile;1,2,3,4,5-pentamethylcyclopenta-1,3-diene;ruthenium(1+);hexafluorophosphate
- Click on QUICK INQUIRY to receive a quote from our team of experts.
- With the quality product at a COMPETITIVE price, you can focus more on your research.
Overview
Description
Acetonitrile;1,2,3,4,5-pentamethylcyclopenta-1,3-diene;ruthenium(1+);hexafluorophosphate is a complex organometallic compound with the molecular formula C16H25F6N3PRu. This compound is known for its unique structure and versatile applications in various scientific fields.
Mechanism of Action
Target of Action
The primary target of Acetonitrile;1,2,3,4,5-pentamethylcyclopenta-1,3-diene;ruthenium(1+);hexafluorophosphate is internal and terminal alkynes . The compound acts as a catalyst for the hydrosilylation of these alkynes .
Mode of Action
This compound interacts with its targets (alkynes) through a process known as hydrosilylation . This interaction results in the transformation of the alkynes .
Biochemical Pathways
The compound affects the hydrosilylation pathway, leading to the transformation of alkynes . The downstream effects include the cycloisomerization of diynes and the synthesis of furans from bis(alkynes) and DMSO .
Result of Action
The molecular and cellular effects of this compound’s action include the transformation of alkynes through hydrosilylation . This leads to the production of new compounds, such as furans .
Biochemical Analysis
Biochemical Properties
Acetonitrile;1,2,3,4,5-pentamethylcyclopenta-1,3-diene;ruthenium(1+);hexafluorophosphate acts as a versatile catalyst in biochemical reactions. It interacts with various enzymes and proteins to facilitate reactions that form carbon-carbon or carbon-heteroatom bonds. The compound’s interaction with enzymes such as hydrosilylation catalysts enables the formation of α-vinylsilanes from alkynes . Additionally, it plays a role in the cyclotrimerization of alkynes, which is essential for synthesizing complex organic molecules .
Cellular Effects
This compound influences various cellular processes. It affects cell signaling pathways, gene expression, and cellular metabolism. The compound’s catalytic properties enable it to participate in reactions that modify cellular components, leading to changes in cell function. For instance, its role in hydrosilylation reactions can impact the synthesis of biomolecules that are crucial for cell signaling and metabolism .
Molecular Mechanism
The molecular mechanism of action of this compound involves its interaction with biomolecules at the molecular level. The compound binds to specific enzymes and proteins, facilitating catalytic reactions that result in the formation of new chemical bonds. This binding interaction can lead to enzyme inhibition or activation, depending on the nature of the reaction. Additionally, the compound can influence gene expression by modifying the activity of transcription factors and other regulatory proteins .
Temporal Effects in Laboratory Settings
In laboratory settings, the effects of this compound can change over time. The compound’s stability and degradation are critical factors that influence its long-term effects on cellular function. Studies have shown that the compound remains stable under specific storage conditions (2-8°C) and can retain its catalytic properties for extended periods . Prolonged exposure to certain environmental conditions may lead to degradation, affecting its efficacy in biochemical reactions .
Dosage Effects in Animal Models
The effects of this compound vary with different dosages in animal models. At lower doses, the compound can effectively catalyze biochemical reactions without causing significant adverse effects. At higher doses, it may exhibit toxic or adverse effects, including enzyme inhibition and disruption of cellular processes . Threshold effects observed in these studies indicate that careful dosage control is essential to maximize the compound’s benefits while minimizing potential risks .
Metabolic Pathways
This compound is involved in various metabolic pathways. It interacts with enzymes and cofactors that facilitate the conversion of substrates into products in biochemical reactions. The compound’s role in hydrosilylation and cyclotrimerization reactions highlights its importance in metabolic flux and the regulation of metabolite levels . These interactions are crucial for maintaining cellular homeostasis and supporting metabolic processes .
Transport and Distribution
The transport and distribution of this compound within cells and tissues are influenced by its interaction with transporters and binding proteins. The compound’s localization and accumulation in specific cellular compartments can affect its activity and function. Studies have shown that the compound can be transported across cell membranes and distributed to various tissues, where it exerts its catalytic effects .
Subcellular Localization
The subcellular localization of this compound is essential for its activity and function. The compound may be directed to specific compartments or organelles through targeting signals or post-translational modifications. Its presence in particular subcellular locations can enhance its catalytic properties and facilitate its interaction with biomolecules involved in biochemical reactions .
Preparation Methods
Synthetic Routes and Reaction Conditions
The synthesis of this compound typically involves the reaction of 1,2,3,4,5-pentamethylcyclopentadiene with acetonitrile in the presence of ruthenium chloride and hexafluorophosphoric acid. The reaction is usually carried out under an inert atmosphere to prevent oxidation and contamination.
Industrial Production Methods
Industrial production of this compound involves scaling up the laboratory synthesis methods. This includes optimizing reaction conditions, such as temperature, pressure, and reaction time, to achieve higher yields and purity. Continuous flow reactors and automated systems are often employed to enhance production efficiency.
Chemical Reactions Analysis
Types of Reactions
This compound undergoes various types of reactions, including:
Oxidation: : The compound can be oxidized to form different oxidation states of ruthenium.
Reduction: : Reduction reactions can be used to alter the oxidation state of ruthenium.
Substitution: : Substitution reactions involve the replacement of ligands in the compound.
Common Reagents and Conditions
Common reagents used in these reactions include oxidizing agents like hydrogen peroxide, reducing agents like sodium borohydride, and various nucleophiles and electrophiles for substitution reactions. Reaction conditions such as temperature, solvent, and reaction time are carefully controlled to achieve the desired products.
Major Products Formed
The major products formed from these reactions include various ruthenium complexes with different ligands, depending on the specific reaction conditions and reagents used.
Scientific Research Applications
This compound has a wide range of applications in scientific research, including:
Catalysis: : It is used as a catalyst in various organic reactions, such as hydrosilylation of alkynes and cycloisomerization of diynes.
Material Science: : The compound is used in the synthesis of advanced materials, including those used in solar energy and water treatment applications.
Biology: : The compound is used in biological studies to understand the interactions between metal complexes and biological molecules.
Comparison with Similar Compounds
This compound is unique due to its specific combination of ligands and the ruthenium center. Similar compounds include other organometallic ruthenium complexes, such as pentamethylcyclopentadienyltris(acetonitrile)ruthenium(II) hexafluorophosphate. These compounds share similarities in their use as catalysts and in material science applications, but the specific ligand environment and oxidation state of ruthenium can lead to different reactivity and properties.
Properties
CAS No. |
99604-67-8 |
---|---|
Molecular Formula |
C16H25F6N3PRu |
Molecular Weight |
505.4 g/mol |
IUPAC Name |
acetonitrile;1,2,3,4,5-pentamethylcyclopenta-1,3-diene;ruthenium(1+);hexafluorophosphate |
InChI |
InChI=1S/C10H16.3C2H3N.F6P.Ru/c1-6-7(2)9(4)10(5)8(6)3;3*1-2-3;1-7(2,3,4,5)6;/h6H,1-5H3;3*1H3;;/q;;;;-1;+1 |
InChI Key |
IYEMDYLFXFMECZ-UHFFFAOYSA-N |
SMILES |
CC#N.CC#N.CC#N.CC1C(=C(C(=C1C)C)C)C.F[P-](F)(F)(F)(F)F.[Ru+] |
Canonical SMILES |
CC#N.CC#N.CC#N.CC1C(=C(C(=C1C)C)C)C.F[P-](F)(F)(F)(F)F.[Ru+] |
Pictograms |
Irritant |
Origin of Product |
United States |
Disclaimer and Information on In-Vitro Research Products
Please be aware that all articles and product information presented on BenchChem are intended solely for informational purposes. The products available for purchase on BenchChem are specifically designed for in-vitro studies, which are conducted outside of living organisms. In-vitro studies, derived from the Latin term "in glass," involve experiments performed in controlled laboratory settings using cells or tissues. It is important to note that these products are not categorized as medicines or drugs, and they have not received approval from the FDA for the prevention, treatment, or cure of any medical condition, ailment, or disease. We must emphasize that any form of bodily introduction of these products into humans or animals is strictly prohibited by law. It is essential to adhere to these guidelines to ensure compliance with legal and ethical standards in research and experimentation.