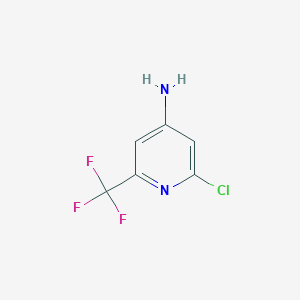
2-Chloro-6-(trifluoromethyl)pyridin-4-amine
Overview
Description
2-Chloro-6-(trifluoromethyl)pyridin-4-amine is a chemical compound with the molecular formula C6H4ClF3N2. It is a derivative of pyridine, a basic heterocyclic organic compound. The presence of both chlorine and trifluoromethyl groups in its structure imparts unique chemical properties, making it valuable in various scientific and industrial applications.
Preparation Methods
Cyclocondensation Reactions Using Trifluoromethyl-Containing Building Blocks
Another key approach to synthesizing trifluoromethylpyridine derivatives involves cyclocondensation reactions starting from trifluoromethylated keto esters or related building blocks.
Key Building Blocks : Ethyl 2,2,2-trifluoroacetate, 2,2,2-trifluoroacetyl chloride, ethyl 4,4,4-trifluoro-3-oxobutanoate, and (E)-4-ethoxy-1,1,1-trifluorobut-3-en-2-one are commonly used as trifluoromethyl sources.
Reaction Mechanism : These building blocks undergo condensation with amines or aldehydes to form trifluoromethyl-substituted pyridine rings, often via intermediate enamine or imine species.
Advantages : This method allows for precise introduction of trifluoromethyl groups at specific positions on the pyridine ring, enabling the synthesis of 2-chloro-6-(trifluoromethyl)pyridin-4-amine after subsequent chlorination steps.
Industrial Relevance : Many agrochemicals containing trifluoromethylpyridine moieties are synthesized via this route, highlighting its robustness and scalability.
A more recent synthetic strategy involves the direct introduction of trifluoromethyl groups onto halopyridines using trifluoromethyl active species such as trifluoromethyl copper reagents.
Methodology : Bromo- or iodopyridines undergo nucleophilic substitution with trifluoromethyl copper reagents to afford trifluoromethylpyridines.
Limitations : This method is generally less common industrially due to the sensitivity of reagents and the need for specialized conditions, but it offers a direct route to trifluoromethylated pyridines without requiring prior functionalization.
Research Status : This approach is primarily reported in academic research and small-scale synthesis rather than large-scale production.
Amination of Chloro(trifluoromethyl)pyridines
To obtain this compound specifically, the amino group at the 4-position can be introduced via nucleophilic aromatic substitution or palladium-catalyzed amination of the corresponding chloro(trifluoromethyl)pyridine.
-
Starting from 2-chloro-6-(trifluoromethyl)pyridine derivatives, amination can be performed using ammonia or amine sources under catalytic conditions.
Palladium-catalyzed Buchwald-Hartwig amination is a common method, employing ligands such as XantPhos and bases like tert-butoxide in toluene at elevated temperatures (~110°C).
-
A mixture of 2-chloro-6-(trifluoromethyl)pyridine, ammonia or amine source, Pd2(dba)3 catalyst, XantPhos ligand, and t-BuONa base in toluene is stirred under nitrogen atmosphere at 110°C for 12 hours.
The reaction mixture is then purified by chromatography or preparative HPLC to isolate the amine product.
Yields and Purity : This method typically affords the desired amine in good yields with high purity, suitable for further synthetic applications.
Summary Table of Preparation Methods
Method | Key Reagents/Conditions | Advantages | Limitations | Typical Yields |
---|---|---|---|---|
Vapor-phase chlorination/fluorination | Picoline derivatives, Cl2, FeF catalyst, >300°C | Industrial scalability, good control of substitution | Multi-chlorinated by-products formation | 60–80% (varies by substrate) |
Cyclocondensation with trifluoromethyl building blocks | Ethyl 2,2,2-trifluoroacetate, trifluoroacetyl chloride | Precise substitution, versatile building blocks | Multi-step synthesis, requires chlorination post-step | Moderate to high |
Direct trifluoromethylation with trifluoromethyl copper | Bromo-/iodopyridines, trifluoromethyl copper reagents | Direct introduction of CF3 group | Sensitive reagents, limited scale | Variable, mostly research scale |
Palladium-catalyzed amination | 2-chloro-6-(trifluoromethyl)pyridine, Pd catalyst, amines | Efficient amination, high purity | Requires expensive catalysts | Good to excellent |
Research Findings and Notes
The vapor-phase chlorination/fluorination method is the most widely used industrial process due to its efficiency and ability to produce key intermediates for agrochemicals such as fluazifop.
Control over reaction parameters allows selective formation of 2-chloro-6-(trifluoromethyl)pyridine derivatives, although some by-product management is necessary.
Cyclocondensation methods provide a flexible synthetic route, especially useful for introducing trifluoromethyl groups at specific ring positions.
Palladium-catalyzed amination is a reliable method for introducing the amino group at the 4-position, completing the synthesis of this compound.
Combining these methods in sequence—starting with vapor-phase synthesis or cyclocondensation, followed by amination—enables efficient preparation of the target compound.
Chemical Reactions Analysis
Types of Reactions
2-Chloro-6-(trifluoromethyl)pyridin-4-amine undergoes various chemical reactions, including:
Substitution Reactions: The chlorine atom can be substituted with other nucleophiles under appropriate conditions.
Oxidation and Reduction: The compound can participate in oxidation and reduction reactions, altering its oxidation state and functional groups.
Coupling Reactions: It can be used in Suzuki–Miyaura coupling reactions to form carbon-carbon bonds.
Common Reagents and Conditions
Substitution: Common reagents include nucleophiles such as amines and thiols.
Oxidation: Oxidizing agents like potassium permanganate or hydrogen peroxide.
Reduction: Reducing agents such as lithium aluminum hydride or sodium borohydride.
Coupling: Palladium catalysts and boron reagents are typically used in Suzuki–Miyaura coupling.
Major Products Formed
The major products formed from these reactions depend on the specific reagents and conditions used. For example, substitution reactions can yield various substituted pyridines, while coupling reactions can produce complex organic molecules with extended carbon chains.
Scientific Research Applications
Chemistry
2-Chloro-6-(trifluoromethyl)pyridin-4-amine serves as an important intermediate in the synthesis of various organic compounds, including pharmaceuticals and agrochemicals. It is utilized in:
- Substitution Reactions : The chlorine atom can be replaced by nucleophiles under suitable conditions.
- Coupling Reactions : It is employed in Suzuki–Miyaura coupling reactions to synthesize complex organic molecules.
Reaction Type | Description | Common Reagents |
---|---|---|
Substitution | Chlorine substitution with nucleophiles | Amines, thiols |
Coupling | Formation of carbon-carbon bonds | Palladium catalysts, boron reagents |
Oxidation/Reduction | Alteration of oxidation states | Potassium permanganate, lithium aluminum hydride |
Biology
The compound's derivatives are studied for their potential biological activities, including:
- Anticancer Activity : Research indicates that it can inhibit specific kinases involved in cancer proliferation, effectively targeting signaling pathways crucial for tumor growth.
- Antimicrobial Properties : Structurally related compounds have shown significant antibacterial activity against strains such as methicillin-resistant Staphylococcus aureus (MRSA). The trifluoromethyl group enhances lipophilicity, aiding membrane penetration.
Medicine
In medicinal chemistry, this compound is a key building block for drug development. Its fluorine content is particularly valuable as fluorinated drugs often exhibit enhanced pharmacokinetic properties. Notable applications include:
- HIV Protease Inhibitors : Compounds derived from this structure are being explored as inhibitors for HIV protease, contributing to antiviral therapies.
Case Studies and Research Findings
- Anticancer Studies : In vitro studies demonstrated that this compound could inhibit cellular proliferation in various cancer cell lines by targeting specific kinases involved in signaling pathways crucial for tumor growth.
- Antimicrobial Testing : Related compounds showed significant antibacterial activity against both Gram-positive and Gram-negative bacteria. These findings suggest potential lead candidates for new antibiotics.
- Receptor Interaction Studies : Modifications to the trifluoromethyl group were found to enhance binding affinity to calcitonin gene-related peptide (CGRP) receptors, indicating potential applications in migraine treatment.
Mechanism of Action
The mechanism of action of 2-Chloro-6-(trifluoromethyl)pyridin-4-amine involves its interaction with specific molecular targets and pathways. The trifluoromethyl group can enhance the compound’s lipophilicity, allowing it to easily penetrate cell membranes and interact with intracellular targets. The chlorine atom can participate in various chemical reactions, modifying the compound’s activity and specificity .
Comparison with Similar Compounds
Similar Compounds
- 2-Chloro-4-(trifluoromethyl)pyridine
- 6-Chloro-4-(trifluoromethyl)pyridin-2-amine
- 2-Chloro-6-(trifluoromethyl)pyridine
Uniqueness
2-Chloro-6-(trifluoromethyl)pyridin-4-amine is unique due to the specific positioning of the chlorine and trifluoromethyl groups on the pyridine ring. This unique structure imparts distinct chemical and physical properties, making it particularly valuable in the synthesis of complex organic molecules and in applications requiring high stability and reactivity .
Biological Activity
2-Chloro-6-(trifluoromethyl)pyridin-4-amine is a pyridine derivative characterized by the presence of a chlorine atom and a trifluoromethyl group at specific positions on the pyridine ring. This unique substitution pattern contributes to its distinct electronic properties, influencing its biological activity. This article explores the compound's biological activity, including its potential therapeutic applications, mechanisms of action, and comparative analysis with similar compounds.
- Molecular Formula : C6H4ClF3N2
- Molecular Weight : 196.56 g/mol
- Structure : The structure can be represented as follows:
Biological Activity Overview
Research indicates that this compound exhibits significant biological activity, particularly in the following areas:
- Anticancer Activity : The compound has been studied for its ability to inhibit specific kinases involved in cancer proliferation. Its interaction with biological targets is enhanced by its electronic structure, allowing effective binding to active sites of enzymes and receptors.
- Antimicrobial Properties : Similar compounds have demonstrated antibacterial activity against strains such as methicillin-resistant Staphylococcus aureus (MRSA). The trifluoromethyl group may enhance lipophilicity, facilitating membrane penetration and enzyme inhibition .
- Receptor Antagonism : It has been noted that derivatives of trifluoromethylpyridines can act as antagonists at calcitonin gene-related peptide (CGRP) receptors, which are implicated in migraine treatment.
The mechanism of action for this compound involves:
- Enzyme Binding : The compound binds to active sites of specific enzymes, modulating biochemical pathways relevant to cancer proliferation and microbial resistance.
- Structural Modifications : Variations in the compound's structure can significantly affect its binding affinity and biological activity, indicating that careful design is crucial for optimizing therapeutic effects.
Comparative Analysis with Similar Compounds
Case Studies and Research Findings
Several studies have highlighted the potential applications of this compound:
- Anticancer Studies : In vitro studies demonstrated that this compound could inhibit cellular proliferation in various cancer cell lines by targeting specific kinases involved in signaling pathways crucial for tumor growth .
- Antimicrobial Testing : Compounds structurally related to this compound showed significant antibacterial activity against both Gram-positive and Gram-negative bacteria. Research indicated that these compounds could serve as lead candidates for developing new antibiotics .
- Receptor Interaction Studies : Investigations into receptor binding revealed that modifications to the trifluoromethyl group could enhance binding affinity to CGRP receptors, suggesting potential applications in migraine treatment.
Q & A
Basic Research Questions
Q. What are the common synthetic routes for preparing 2-Chloro-6-(trifluoromethyl)pyridin-4-amine, and how are intermediates characterized?
The synthesis typically involves functionalization of pyridine precursors. For example, tert-butyl carbamate intermediates (e.g., tert-butyl (2-chloro-6-(trifluoromethyl)pyridin-4-yl)carbamate) are prepared via nucleophilic substitution or coupling reactions. Key intermediates are characterized using LC-MS (e.g., m/z 240 [M+H-tBu]+), 1H-NMR (δ 8.01–8.68 ppm for aromatic protons), and X-ray crystallography to confirm regiochemistry and purity .
Q. How is the purity and structural identity of this compound validated in academic research?
Purity is assessed via HPLC (≥98% purity criteria) and LC-MS to detect trace impurities. Structural confirmation employs 1H/13C-NMR (aromatic proton splitting patterns, CF3 group signals) and FT-IR (C-Cl and C-F stretching vibrations). For crystalline derivatives, SHELX -based refinement of X-ray diffraction data ensures accurate bond-length and angle measurements .
Q. What spectroscopic techniques are critical for analyzing substituent effects in this compound?
- 19F-NMR quantifies electronic effects of the trifluoromethyl group.
- UV-Vis spectroscopy evaluates conjugation effects in derivatives.
- DSC/TGA analyzes thermal stability, critical for reaction condition optimization .
Advanced Research Questions
Q. How can structural ambiguities in derivatives (e.g., regioisomers) be resolved experimentally?
- X-ray crystallography (using SHELXL for refinement) provides unambiguous spatial assignments.
- NOESY/ROESY NMR detects through-space interactions to distinguish substituent positions.
- DFT calculations (e.g., Gaussian) predict spectroscopic profiles for comparison with experimental data .
Q. What strategies mitigate conflicting bioactivity data between in vitro and in vivo studies for this compound?
- Metabolic stability assays (e.g., liver microsomes) identify rapid degradation pathways.
- Pharmacokinetic profiling (Cmax, AUC) clarifies bioavailability limitations.
- Cryo-EM or crystallography of target-ligand complexes validates binding modes observed in vitro .
Q. How does the trifluoromethyl group influence reaction mechanisms in cross-coupling reactions?
The -CF3 group exerts strong electron-withdrawing effects , directing electrophilic substitution to the para position. In Suzuki-Miyaura couplings, it stabilizes transition states via inductive effects, as shown by kinetic isotope effect (KIE) studies and Hammett plots .
Q. What computational methods predict the compound’s interaction with biological targets (e.g., LOXL2)?
- Molecular docking (AutoDock Vina) identifies binding poses in enzyme active sites.
- MD simulations (AMBER/CHARMM) assess binding stability over time.
- QSAR models correlate substituent electronic parameters (Hammett σ) with inhibitory potency .
Q. How are reactive intermediates (e.g., propargylamine derivatives) characterized during synthesis?
- In situ FT-IR monitors reaction progress by tracking carbonyl or alkyne peaks.
- HRMS (High-Resolution Mass Spectrometry) confirms intermediate masses.
- Stopped-flow NMR captures transient species in fast reactions .
Q. What experimental approaches optimize regioselectivity in nucleophilic substitution reactions?
- Solvent polarity screening (e.g., DMF vs. THF) alters transition-state stabilization.
- Lewis acid catalysts (e.g., ZnCl2) coordinate with leaving groups to direct attack.
- Isotopic labeling (e.g., 15N) tracks substitution pathways via NMR .
Q. How are SAR studies designed to enhance selectivity for enzyme targets (e.g., LOXL2 over LOX)?
- Alanine scanning mutagenesis identifies key residues for selectivity.
- Fluorine scanning introduces -F/-CF3 groups to exploit hydrophobic pockets.
- Kinetic assays (kcat/KM) quantify inhibitor potency and isoform specificity .
Q. Methodological Notes
- Data Contradictions : Conflicting crystallographic vs. spectroscopic data require Bader charge analysis (AIM theory) to reconcile electronic effects .
- Reaction Optimization : Use DoE (Design of Experiments) to statistically optimize yield and regioselectivity .
- Biological Assays : Include counter-screens against related enzymes (e.g., MAO-A/B) to validate specificity .
Properties
IUPAC Name |
2-chloro-6-(trifluoromethyl)pyridin-4-amine | |
---|---|---|
Source | PubChem | |
URL | https://pubchem.ncbi.nlm.nih.gov | |
Description | Data deposited in or computed by PubChem | |
InChI |
InChI=1S/C6H4ClF3N2/c7-5-2-3(11)1-4(12-5)6(8,9)10/h1-2H,(H2,11,12) | |
Source | PubChem | |
URL | https://pubchem.ncbi.nlm.nih.gov | |
Description | Data deposited in or computed by PubChem | |
InChI Key |
MXOHYUGYDQGRGI-UHFFFAOYSA-N | |
Source | PubChem | |
URL | https://pubchem.ncbi.nlm.nih.gov | |
Description | Data deposited in or computed by PubChem | |
Canonical SMILES |
C1=C(C=C(N=C1C(F)(F)F)Cl)N | |
Source | PubChem | |
URL | https://pubchem.ncbi.nlm.nih.gov | |
Description | Data deposited in or computed by PubChem | |
Molecular Formula |
C6H4ClF3N2 | |
Source | PubChem | |
URL | https://pubchem.ncbi.nlm.nih.gov | |
Description | Data deposited in or computed by PubChem | |
DSSTOX Substance ID |
DTXSID80558392 | |
Record name | 2-Chloro-6-(trifluoromethyl)pyridin-4-amine | |
Source | EPA DSSTox | |
URL | https://comptox.epa.gov/dashboard/DTXSID80558392 | |
Description | DSSTox provides a high quality public chemistry resource for supporting improved predictive toxicology. | |
Molecular Weight |
196.56 g/mol | |
Source | PubChem | |
URL | https://pubchem.ncbi.nlm.nih.gov | |
Description | Data deposited in or computed by PubChem | |
CAS No. |
34486-22-1 | |
Record name | 2-Chloro-6-(trifluoromethyl)pyridin-4-amine | |
Source | EPA DSSTox | |
URL | https://comptox.epa.gov/dashboard/DTXSID80558392 | |
Description | DSSTox provides a high quality public chemistry resource for supporting improved predictive toxicology. | |
Retrosynthesis Analysis
AI-Powered Synthesis Planning: Our tool employs the Template_relevance Pistachio, Template_relevance Bkms_metabolic, Template_relevance Pistachio_ringbreaker, Template_relevance Reaxys, Template_relevance Reaxys_biocatalysis model, leveraging a vast database of chemical reactions to predict feasible synthetic routes.
One-Step Synthesis Focus: Specifically designed for one-step synthesis, it provides concise and direct routes for your target compounds, streamlining the synthesis process.
Accurate Predictions: Utilizing the extensive PISTACHIO, BKMS_METABOLIC, PISTACHIO_RINGBREAKER, REAXYS, REAXYS_BIOCATALYSIS database, our tool offers high-accuracy predictions, reflecting the latest in chemical research and data.
Strategy Settings
Precursor scoring | Relevance Heuristic |
---|---|
Min. plausibility | 0.01 |
Model | Template_relevance |
Template Set | Pistachio/Bkms_metabolic/Pistachio_ringbreaker/Reaxys/Reaxys_biocatalysis |
Top-N result to add to graph | 6 |
Feasible Synthetic Routes
Disclaimer and Information on In-Vitro Research Products
Please be aware that all articles and product information presented on BenchChem are intended solely for informational purposes. The products available for purchase on BenchChem are specifically designed for in-vitro studies, which are conducted outside of living organisms. In-vitro studies, derived from the Latin term "in glass," involve experiments performed in controlled laboratory settings using cells or tissues. It is important to note that these products are not categorized as medicines or drugs, and they have not received approval from the FDA for the prevention, treatment, or cure of any medical condition, ailment, or disease. We must emphasize that any form of bodily introduction of these products into humans or animals is strictly prohibited by law. It is essential to adhere to these guidelines to ensure compliance with legal and ethical standards in research and experimentation.