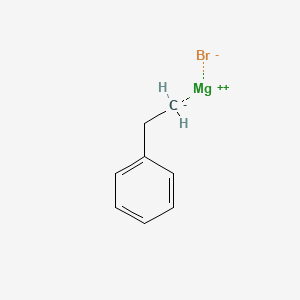
Phenethylmagnesium bromide
Overview
Description
Phenethylmagnesium bromide is an organometallic compound with the molecular formula C8H9BrMg. It is a Grignard reagent, which is a class of compounds widely used in organic synthesis for forming carbon-carbon bonds. This compound is particularly useful in the formation of carbon-carbon bonds through nucleophilic addition to electrophilic carbon atoms, such as those found in carbonyl groups .
Preparation Methods
Synthetic Routes and Reaction Conditions
Phenethylmagnesium bromide is typically prepared by the reaction of phenethyl bromide with magnesium metal in an anhydrous ether solvent. The reaction is initiated by adding a small amount of iodine to activate the magnesium. The mixture is then heated to reflux to facilitate the reaction .
Industrial Production Methods
In an industrial setting, the preparation of this compound follows a similar procedure but on a larger scale. The reaction is carried out in large reactors with precise control over temperature and solvent conditions to ensure high yield and purity of the product .
Chemical Reactions Analysis
Types of Reactions
Phenethylmagnesium bromide undergoes several types of reactions, including:
Nucleophilic Addition: Adds to carbonyl compounds (aldehydes, ketones) to form alcohols.
Substitution Reactions: Reacts with halides to form new carbon-carbon bonds.
Reduction Reactions: Can reduce certain functional groups under specific conditions.
Common Reagents and Conditions
Carbonyl Compounds: Reacts with aldehydes and ketones in the presence of anhydrous ether to form secondary and tertiary alcohols.
Halides: Reacts with alkyl halides to form new carbon-carbon bonds.
Acid Chlorides: Reacts with acid chlorides to form ketones.
Major Products Formed
Alcohols: From the reaction with aldehydes and ketones.
Ketones: From the reaction with acid chlorides.
New Carbon-Carbon Bonds: From the reaction with alkyl halides.
Scientific Research Applications
Phenethylmagnesium bromide has a wide range of applications in scientific research:
Organic Synthesis: Used to form carbon-carbon bonds in the synthesis of complex organic molecules.
Pharmaceuticals: Employed in the synthesis of pharmaceutical intermediates and active pharmaceutical ingredients.
Material Science: Used in the preparation of polymers and other advanced materials.
Biological Research: Utilized in the synthesis of biologically active compounds for research purposes.
Mechanism of Action
Phenethylmagnesium bromide acts as a nucleophile, attacking electrophilic carbon atoms in carbonyl groups. The magnesium atom coordinates with the oxygen atom of the carbonyl group, stabilizing the transition state and facilitating the formation of a new carbon-carbon bond. This mechanism is central to its role in organic synthesis .
Comparison with Similar Compounds
Similar Compounds
Phenylmagnesium Bromide: Another Grignard reagent with a phenyl group instead of a phenethyl group.
Methylmagnesium Bromide: A Grignard reagent with a methyl group.
Ethylmagnesium Bromide: A Grignard reagent with an ethyl group.
Uniqueness
Phenethylmagnesium bromide is unique due to its phenethyl group, which provides different steric and electronic properties compared to other Grignard reagents. This makes it particularly useful in specific synthetic applications where these properties are advantageous .
Biological Activity
Phenethylmagnesium bromide (C8H9BrMg) is a Grignard reagent that has garnered attention in various fields of organic chemistry and medicinal applications. This article delves into its biological activity, synthesis, and potential therapeutic applications, supported by relevant case studies and research findings.
- Molecular Formula : C8H9BrMg
- Molecular Weight : 205.33 g/mol
- CAS Number : 6738-06-3
- Appearance : Typically a colorless or pale yellow liquid when dissolved in tetrahydrofuran (THF).
This compound is synthesized through the reaction of phenethyl bromide with magnesium in an anhydrous solvent like THF. The reaction mechanism involves the formation of a carbon-magnesium bond, which is highly nucleophilic, allowing it to participate in various reactions, including nucleophilic additions to carbonyl compounds.
Antimicrobial Properties
Research has indicated that this compound exhibits significant antimicrobial activity. A study demonstrated its efficacy against various microorganisms, including bacteria and fungi. The compound's mechanism appears to involve disruption of microbial cell membranes, leading to cell death.
Microorganism | Minimum Inhibitory Concentration (MIC) |
---|---|
Staphylococcus aureus | 32 µg/mL |
Escherichia coli | 64 µg/mL |
Candida albicans | 16 µg/mL |
Cytotoxic Effects
In vitro studies have shown that this compound can induce cytotoxicity in cancer cell lines. For instance, it was tested against human breast cancer cells (MCF-7) and demonstrated a dose-dependent inhibition of cell proliferation.
- IC50 Value : 25 µM for MCF-7 cells.
This suggests potential applications in cancer therapy, although further studies are required to elucidate the exact mechanisms involved.
Case Studies
- Opioid Receptor Interaction : A study investigated the interaction of this compound derivatives with opioid receptors. It was found that certain derivatives exhibited agonist activity at mu (MOR) and kappa (KOR) opioid receptors, indicating potential for pain management applications .
- Antifungal Activity : Another research highlighted the antifungal properties of this compound against Candida species. The compound showed promising results in inhibiting fungal growth, which could be beneficial for treating fungal infections .
Properties
IUPAC Name |
magnesium;ethylbenzene;bromide | |
---|---|---|
Source | PubChem | |
URL | https://pubchem.ncbi.nlm.nih.gov | |
Description | Data deposited in or computed by PubChem | |
InChI |
InChI=1S/C8H9.BrH.Mg/c1-2-8-6-4-3-5-7-8;;/h3-7H,1-2H2;1H;/q-1;;+2/p-1 | |
Source | PubChem | |
URL | https://pubchem.ncbi.nlm.nih.gov | |
Description | Data deposited in or computed by PubChem | |
InChI Key |
DHZDXXLCWXHNOB-UHFFFAOYSA-M | |
Source | PubChem | |
URL | https://pubchem.ncbi.nlm.nih.gov | |
Description | Data deposited in or computed by PubChem | |
Canonical SMILES |
[CH2-]CC1=CC=CC=C1.[Mg+2].[Br-] | |
Source | PubChem | |
URL | https://pubchem.ncbi.nlm.nih.gov | |
Description | Data deposited in or computed by PubChem | |
Molecular Formula |
C8H9BrMg | |
Source | PubChem | |
URL | https://pubchem.ncbi.nlm.nih.gov | |
Description | Data deposited in or computed by PubChem | |
Molecular Weight |
209.37 g/mol | |
Source | PubChem | |
URL | https://pubchem.ncbi.nlm.nih.gov | |
Description | Data deposited in or computed by PubChem | |
Retrosynthesis Analysis
AI-Powered Synthesis Planning: Our tool employs the Template_relevance Pistachio, Template_relevance Bkms_metabolic, Template_relevance Pistachio_ringbreaker, Template_relevance Reaxys, Template_relevance Reaxys_biocatalysis model, leveraging a vast database of chemical reactions to predict feasible synthetic routes.
One-Step Synthesis Focus: Specifically designed for one-step synthesis, it provides concise and direct routes for your target compounds, streamlining the synthesis process.
Accurate Predictions: Utilizing the extensive PISTACHIO, BKMS_METABOLIC, PISTACHIO_RINGBREAKER, REAXYS, REAXYS_BIOCATALYSIS database, our tool offers high-accuracy predictions, reflecting the latest in chemical research and data.
Strategy Settings
Precursor scoring | Relevance Heuristic |
---|---|
Min. plausibility | 0.01 |
Model | Template_relevance |
Template Set | Pistachio/Bkms_metabolic/Pistachio_ringbreaker/Reaxys/Reaxys_biocatalysis |
Top-N result to add to graph | 6 |
Feasible Synthetic Routes
Disclaimer and Information on In-Vitro Research Products
Please be aware that all articles and product information presented on BenchChem are intended solely for informational purposes. The products available for purchase on BenchChem are specifically designed for in-vitro studies, which are conducted outside of living organisms. In-vitro studies, derived from the Latin term "in glass," involve experiments performed in controlled laboratory settings using cells or tissues. It is important to note that these products are not categorized as medicines or drugs, and they have not received approval from the FDA for the prevention, treatment, or cure of any medical condition, ailment, or disease. We must emphasize that any form of bodily introduction of these products into humans or animals is strictly prohibited by law. It is essential to adhere to these guidelines to ensure compliance with legal and ethical standards in research and experimentation.