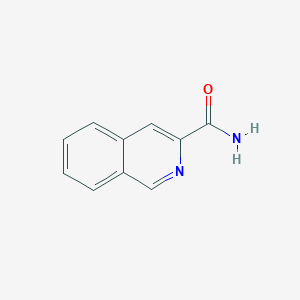
Isoquinoline-3-carboxamide
Overview
Description
Isoquinoline-3-carboxamide is a heterocyclic aromatic compound that features an isoquinoline core with a carboxamide group at the third position. This compound is of significant interest in medicinal chemistry due to its potential therapeutic applications, particularly in the treatment of autoimmune and inflammatory diseases .
Mechanism of Action
Target of Action
Isoquinoline-3-carboxamide has been found to primarily target S100A9 , a molecule expressed on the surface of various monocyte populations in the peripheral blood . It also interacts with natural killer (NK) cells and dendritic cells (DCs) .
Mode of Action
This compound interacts with its targets in a complex manner. It binds to S100A9, inhibiting the interaction of S100A9 with two well-known proinflammatory receptors, the Toll-like receptor 4 (TLR4) and receptor of advanced glycation end products (RAGE) . This compound also activates NK cells via the aryl hydrocarbon receptor and increases their DNAX accessory molecule-1 (DNAM-1) cell surface expression .
Biochemical Pathways
The activation of NK cells by this compound improves the cytotoxicity of NK cells against certain types of cells, such as B16F10 melanoma cells . This activation also augments their immunoregulatory functions in certain conditions by interacting with CD155+ dendritic cells (DCs) .
Pharmacokinetics
Studies on similar compounds, such as paquinimod, have shown that these compounds can be efficacious and safe at certain doses .
Result of Action
The result of this compound’s action is a decrease in inflammation and a regression of fibrosis in certain conditions, even when the treatment is initiated after the onset of disease . This is associated with a systemic decrease in the number and reduced activation of disease-promoting transgenic natural killer T (NKT)-II cells and their type 2-cytokine expression profile .
Biochemical Analysis
Biochemical Properties
Isoquinoline-3-carboxamide plays a crucial role in various biochemical reactions. It interacts with several enzymes, proteins, and other biomolecules. For instance, it has been shown to bind to the human S100A9 protein, a key player in inflammatory processes . This interaction is dependent on the presence of zinc and calcium ions. This compound also inhibits the interaction of S100A9 with proinflammatory receptors such as the Toll-like receptor 4 (TLR4) and the receptor for advanced glycation end products (RAGE) . These interactions highlight the compound’s potential as an anti-inflammatory agent.
Cellular Effects
This compound exerts significant effects on various cell types and cellular processes. It has been shown to activate natural killer (NK) cells via the aryl hydrocarbon receptor, enhancing their cytotoxicity against tumor cells and their immunoregulatory functions . This activation leads to increased expression of DNAX accessory molecule-1 (DNAM-1) on the surface of NK cells, which is crucial for their interaction with dendritic cells . Additionally, this compound influences cell signaling pathways, gene expression, and cellular metabolism, contributing to its anti-inflammatory and anti-tumor properties .
Molecular Mechanism
The molecular mechanism of action of this compound involves several key interactions. It binds to the S100A9 protein, inhibiting its interaction with TLR4 and RAGE, which are involved in proinflammatory signaling pathways . This binding reduces the release of proinflammatory cytokines such as tumor necrosis factor-alpha (TNF-α). This compound also activates NK cells through the aryl hydrocarbon receptor, leading to increased DNAM-1 expression and enhanced NK cell function . These molecular interactions underpin the compound’s immunomodulatory and anti-inflammatory effects.
Temporal Effects in Laboratory Settings
In laboratory settings, the effects of this compound have been observed to change over time. The compound is relatively stable, but its long-term effects on cellular function can vary. For example, prolonged exposure to this compound has been shown to sustain the activation of NK cells and maintain their enhanced cytotoxicity . The stability and degradation of the compound in different experimental conditions can influence its efficacy and potency.
Dosage Effects in Animal Models
The effects of this compound vary with different dosages in animal models. At lower doses, the compound effectively reduces inflammation and modulates immune responses without significant adverse effects . At higher doses, this compound can exhibit toxic effects, including liver toxicity and immunosuppression . These dosage-dependent effects highlight the importance of optimizing the dosage for therapeutic applications.
Metabolic Pathways
This compound is involved in several metabolic pathways. It interacts with enzymes such as acetyl-CoA carboxylase and fatty acid synthase, influencing lipid metabolism . The compound also affects the levels of various metabolites, including those involved in energy production and cellular respiration . These interactions suggest that this compound can modulate metabolic flux and contribute to its therapeutic effects.
Transport and Distribution
Within cells and tissues, this compound is transported and distributed through interactions with specific transporters and binding proteins. For instance, it has been shown to interact with the aryl hydrocarbon receptor, facilitating its transport into cells . The compound’s distribution within tissues can affect its localization and accumulation, influencing its overall efficacy and potency.
Subcellular Localization
This compound is localized to specific subcellular compartments, which can affect its activity and function. For example, it has been found to associate with the mitochondrial outer membrane, where it interacts with the peripheral-type benzodiazepine receptor . This localization is crucial for its role in modulating mitochondrial function and cellular metabolism. Additionally, this compound may undergo post-translational modifications that direct it to specific organelles, further influencing its biochemical properties and therapeutic potential.
Preparation Methods
Synthetic Routes and Reaction Conditions: Isoquinoline-3-carboxamide can be synthesized through various methods. One common approach involves the Pomeranz-Fritsch reaction, which entails the condensation of benzaldehyde with aminoacetaldehyde diethyl acetal in an acidic medium . Another method is the Bischler-Napieralski reaction, which involves the cyclization of β-phenylethylamine derivatives in the presence of a dehydrating agent .
Industrial Production Methods: Industrial production of this compound typically involves large-scale synthesis using optimized versions of the aforementioned methods. The choice of method depends on factors such as yield, purity, and cost-effectiveness. Transition metal-catalyzed reactions and green chemistry approaches are also explored to enhance efficiency and reduce environmental impact .
Chemical Reactions Analysis
Types of Reactions: Isoquinoline-3-carboxamide undergoes various chemical reactions, including:
Reduction: Reduction reactions can convert this compound to its corresponding amine derivatives.
Substitution: Electrophilic and nucleophilic substitution reactions are common, given the aromatic nature of the isoquinoline ring.
Common Reagents and Conditions:
Oxidation: Potassium permanganate (KMnO4) in an alkaline medium.
Reduction: Hydrogenation using palladium on carbon (Pd/C) as a catalyst.
Substitution: Various electrophiles and nucleophiles under acidic or basic conditions.
Major Products:
Oxidation: Pyridine-3,4-dicarboxylic acid and its anhydride.
Reduction: Amine derivatives.
Substitution: Substituted isoquinoline derivatives.
Scientific Research Applications
Isoquinoline-3-carboxamide has a wide range of scientific research applications:
Comparison with Similar Compounds
Isoquinoline-3-carboxamide can be compared with other similar compounds, such as:
Quinoline-3-carboxamide: Both compounds share a similar core structure but differ in the position of the carboxamide group.
Chloroquine: Although primarily an antimalarial drug, chloroquine shares the quinoline core structure and exhibits immunomodulatory effects.
Uniqueness: this compound is unique due to its specific interaction with the S100A9 protein and its potential to inhibit proinflammatory pathways, making it a promising candidate for the treatment of autoimmune diseases .
Properties
IUPAC Name |
isoquinoline-3-carboxamide | |
---|---|---|
Source | PubChem | |
URL | https://pubchem.ncbi.nlm.nih.gov | |
Description | Data deposited in or computed by PubChem | |
InChI |
InChI=1S/C10H8N2O/c11-10(13)9-5-7-3-1-2-4-8(7)6-12-9/h1-6H,(H2,11,13) | |
Source | PubChem | |
URL | https://pubchem.ncbi.nlm.nih.gov | |
Description | Data deposited in or computed by PubChem | |
InChI Key |
KKSZSACCVDIWIE-UHFFFAOYSA-N | |
Source | PubChem | |
URL | https://pubchem.ncbi.nlm.nih.gov | |
Description | Data deposited in or computed by PubChem | |
Canonical SMILES |
C1=CC=C2C=NC(=CC2=C1)C(=O)N | |
Source | PubChem | |
URL | https://pubchem.ncbi.nlm.nih.gov | |
Description | Data deposited in or computed by PubChem | |
Molecular Formula |
C10H8N2O | |
Source | PubChem | |
URL | https://pubchem.ncbi.nlm.nih.gov | |
Description | Data deposited in or computed by PubChem | |
DSSTOX Substance ID |
DTXSID90565532 | |
Record name | Isoquinoline-3-carboxamide | |
Source | EPA DSSTox | |
URL | https://comptox.epa.gov/dashboard/DTXSID90565532 | |
Description | DSSTox provides a high quality public chemistry resource for supporting improved predictive toxicology. | |
Molecular Weight |
172.18 g/mol | |
Source | PubChem | |
URL | https://pubchem.ncbi.nlm.nih.gov | |
Description | Data deposited in or computed by PubChem | |
CAS No. |
50458-77-0 | |
Record name | Isoquinoline-3-carboxamide | |
Source | EPA DSSTox | |
URL | https://comptox.epa.gov/dashboard/DTXSID90565532 | |
Description | DSSTox provides a high quality public chemistry resource for supporting improved predictive toxicology. | |
Retrosynthesis Analysis
AI-Powered Synthesis Planning: Our tool employs the Template_relevance Pistachio, Template_relevance Bkms_metabolic, Template_relevance Pistachio_ringbreaker, Template_relevance Reaxys, Template_relevance Reaxys_biocatalysis model, leveraging a vast database of chemical reactions to predict feasible synthetic routes.
One-Step Synthesis Focus: Specifically designed for one-step synthesis, it provides concise and direct routes for your target compounds, streamlining the synthesis process.
Accurate Predictions: Utilizing the extensive PISTACHIO, BKMS_METABOLIC, PISTACHIO_RINGBREAKER, REAXYS, REAXYS_BIOCATALYSIS database, our tool offers high-accuracy predictions, reflecting the latest in chemical research and data.
Strategy Settings
Precursor scoring | Relevance Heuristic |
---|---|
Min. plausibility | 0.01 |
Model | Template_relevance |
Template Set | Pistachio/Bkms_metabolic/Pistachio_ringbreaker/Reaxys/Reaxys_biocatalysis |
Top-N result to add to graph | 6 |
Feasible Synthetic Routes
A: Isoquinoline-3-carboxamide derivatives, specifically those structurally similar to PK11195 (1-(2-chlorophenyl)-N-methyl-N-(1-methylpropyl)this compound), exhibit high affinity for the translocator protein (TSPO), formerly known as the peripheral benzodiazepine receptor (PBR) [, , , , , ]. TSPO is primarily located in the outer mitochondrial membrane and is involved in various cellular processes, including cholesterol transport, steroidogenesis, and apoptosis. While the exact mechanism of action of Isoquinoline-3-carboxamides like PK11195 is not fully elucidated, their binding to TSPO is thought to modulate these downstream processes, influencing cellular responses in various tissues, including the brain [, ].
A: The molecular formula of PK11195 (1-(2-chlorophenyl)-N-methyl-N-(1-methylpropyl)this compound) is C21H21ClN2O, and its molecular weight is 352.86 g/mol [].
ANone: The provided research papers primarily focus on the synthesis, radiolabeling, and biological evaluation of this compound derivatives. They do not provide detailed information on their material compatibility or stability under various conditions. Further research is needed to explore these aspects.
ANone: The provided research focuses on the use of Isoquinoline-3-carboxamides as ligands for TSPO and their potential as therapeutic agents or imaging probes. There is no mention of catalytic properties or applications based on catalysis for this class of compounds.
A: While the provided research does not explicitly detail computational studies on Isoquinoline-3-carboxamides, it highlights the importance of structure-activity relationships (SAR) for this class of compounds []. Computational chemistry tools, such as molecular docking and quantitative structure-activity relationship (QSAR) modeling, are valuable for investigating SAR and optimizing compound properties. Such approaches could be applied to Isoquinoline-3-carboxamides to guide the design of novel derivatives with improved potency, selectivity, or pharmacokinetic profiles.
A: Research on Isoquinoline-3-carboxamides, particularly those targeting TSPO, highlights the impact of structural modifications on their binding affinity and selectivity [, , ]. For example, modifications to the substituents on the isoquinoline ring, the carboxamide group, and the aryl group can significantly alter their pharmacological profile. These modifications can affect their interaction with TSPO, influencing their ability to modulate downstream cellular processes.
ANone: While the provided research primarily focuses on the scientific aspects of Isoquinoline-3-carboxamides, it's crucial to acknowledge the importance of SHE regulations throughout their research and development. This includes adhering to appropriate handling procedures, waste disposal protocols, and risk assessments to minimize potential hazards to human health and the environment.
A: The research on Isoquinoline-3-carboxamides provides some insights into their PK/PD properties. Studies using radiolabeled PK11195 analogues in rats indicate uptake in organs such as the adrenal glands, heart, lung, and kidneys []. This suggests distribution to these tissues, while the observed blocking effect of cold PK11195 highlights specific binding to the target. Additionally, research on saquinavir, a compound with a decahydrothis compound moiety, sheds light on potential metabolic pathways [, ]. The identification of α-hydroxyaldehyde formation through N-dealkylation, primarily mediated by CYP3A enzymes, provides valuable information for understanding the potential metabolism of related Isoquinoline-3-carboxamides. Further research is needed to fully elucidate the ADME profile of different derivatives in various species.
A: Research utilizing this compound derivatives, particularly radiolabeled PK11195, demonstrates their ability to visualize and quantify neuroinflammation in preclinical models [, , ]. For instance, in a rat model of acute neuroinflammation induced by intrastriatal injection of (R,S)-α-amino-3-hydroxy-5-methyl-4-isoxazolopropionique, both [18F]DPA-714 and [11C]PK11195 successfully localized the neuroinflammatory site, with [18F]DPA-714 demonstrating superior performance due to its higher bioavailability and lower nonspecific binding []. Additionally, in experimental autoimmune encephalomyelitis (EAE) rats, a model for multiple sclerosis, [18F]DPA-714 enabled PET imaging of neuroinflammation in the spinal cord, reflecting the increased TSPO expression associated with microglial activation []. These findings highlight the potential of Isoquinoline-3-carboxamides as valuable tools for monitoring neuroinflammatory processes in vivo.
Disclaimer and Information on In-Vitro Research Products
Please be aware that all articles and product information presented on BenchChem are intended solely for informational purposes. The products available for purchase on BenchChem are specifically designed for in-vitro studies, which are conducted outside of living organisms. In-vitro studies, derived from the Latin term "in glass," involve experiments performed in controlled laboratory settings using cells or tissues. It is important to note that these products are not categorized as medicines or drugs, and they have not received approval from the FDA for the prevention, treatment, or cure of any medical condition, ailment, or disease. We must emphasize that any form of bodily introduction of these products into humans or animals is strictly prohibited by law. It is essential to adhere to these guidelines to ensure compliance with legal and ethical standards in research and experimentation.