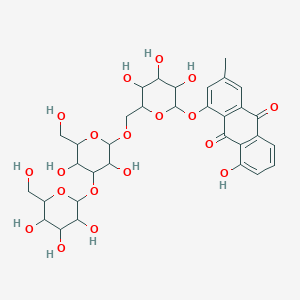
Chrysophanol 1-triglucoside
Overview
Description
Chrysophanol 1-triglucoside is a naturally occurring anthraquinone derivative found in various plants, including Cassia obtusifolia. It is known for its broad-spectrum therapeutic potential and ecological importance. This compound has been studied for its pharmacological properties, including anticancer, hepatoprotective, neuroprotective, anti-inflammatory, antiulcer, and antimicrobial activities .
Mechanism of Action
Target of Action
Chrysophanol 1-triglucoside, also known as 1-[6-[[3,5-dihydroxy-6-(hydroxymethyl)-4-[3,4,5-trihydroxy-6-(hydroxymethyl)oxan-2-yl]oxyoxan-2-yl]oxymethyl]-3,4,5-trihydroxyoxan-2-yl]oxy-8-hydroxy-3-methylanthracene-9,10-dione, primarily targets protein tyrosine phosphatases 1B (PTP1B) and α-glucosidase . These targets play a significant role in metabolic processes, with PTP1B being involved in insulin signaling and α-glucosidase in carbohydrate digestion .
Mode of Action
This compound interacts with its targets by inhibiting their activity. It inhibits PTP1B and α-glucosidase with IC50 values of 80.17 µM and 197.06 µM, respectively . This inhibition can lead to changes in the metabolic processes regulated by these enzymes.
Biochemical Pathways
The inhibition of PTP1B and α-glucosidase by this compound affects several biochemical pathways. The inhibition of PTP1B can enhance insulin signaling, which is crucial for glucose metabolism . On the other hand, the inhibition of α-glucosidase can slow down carbohydrate digestion, reducing the absorption of glucose . These effects can have downstream impacts on energy metabolism and blood glucose levels.
Pharmacokinetics
The compound’s ability to inhibit ptp1b and α-glucosidase suggests that it can be absorbed and reach its targets in the body .
Result of Action
The molecular and cellular effects of this compound’s action include enhanced insulin signaling due to PTP1B inhibition and reduced glucose absorption due to α-glucosidase inhibition . These effects can potentially help in managing conditions like diabetes .
Biochemical Analysis
Biochemical Properties
Chrysophanol 1-triglucoside has been found to inhibit protein tyrosine phosphatases 1B (PTP1B) and α-glucosidase . The inhibition of PTP1B can have significant implications in the regulation of insulin signaling, as PTP1B is a key target of this pathway .
Cellular Effects
In cellular models, this compound has shown significant effects. For instance, it has been reported to inhibit the progression of colorectal cancer by targeting the KITENIN/ErbB4 complex . It disrupts the ErbB4/KITENIN complex, causing autophagic degradation of KITENIN .
Preparation Methods
Synthetic Routes and Reaction Conditions: Chrysophanol 1-triglucoside can be synthesized through the glycosylation of chrysophanol. The reaction typically involves the use of a glycosyl donor, such as a trichloroacetimidate or a thioglycoside, in the presence of a Lewis acid catalyst like boron trifluoride etherate. The reaction is carried out under anhydrous conditions at low temperatures to ensure high yield and selectivity .
Industrial Production Methods: Industrial production of this compound involves the extraction of chrysophanol from plant sources followed by enzymatic glycosylation. The process includes the use of glycosyltransferases to transfer glucose units to chrysophanol, forming this compound. This method is preferred for its efficiency and eco-friendliness .
Chemical Reactions Analysis
Types of Reactions: Chrysophanol 1-triglucoside undergoes various chemical reactions, including:
Oxidation: this compound can be oxidized to form this compound quinone.
Reduction: Reduction of this compound leads to the formation of dihydrothis compound.
Substitution: Nucleophilic substitution reactions can occur at the hydroxyl groups of the glucose moieties.
Common Reagents and Conditions:
Oxidation: Common oxidizing agents include potassium permanganate and hydrogen peroxide.
Reduction: Reducing agents such as sodium borohydride and lithium aluminum hydride are used.
Substitution: Nucleophiles like alkoxides and amines are employed under basic conditions.
Major Products Formed:
Oxidation: this compound quinone.
Reduction: Dihydrothis compound.
Substitution: Various substituted this compound derivatives.
Scientific Research Applications
Chrysophanol 1-triglucoside has a wide range of scientific research applications:
Chemistry: Used as a precursor for the synthesis of other anthraquinone derivatives.
Medicine: Investigated for its anticancer, neuroprotective, and anti-inflammatory properties.
Industry: Utilized in the development of natural dyes and pigments due to its anthraquinone structure.
Comparison with Similar Compounds
Chrysophanol 1-triglucoside is unique compared to other anthraquinone derivatives due to its glycosylation, which enhances its solubility and bioavailability. Similar compounds include:
Chrysophanol: The aglycone form of this compound, known for its similar pharmacological properties but lower solubility.
Aloe-emodin: Similar to chrysophanol, but primarily found in Aloe species and used for its laxative effects.
This compound stands out due to its enhanced solubility and potential for diabetes research, making it a valuable compound in both scientific research and industrial applications .
Properties
IUPAC Name |
1-[6-[[3,5-dihydroxy-6-(hydroxymethyl)-4-[3,4,5-trihydroxy-6-(hydroxymethyl)oxan-2-yl]oxyoxan-2-yl]oxymethyl]-3,4,5-trihydroxyoxan-2-yl]oxy-8-hydroxy-3-methylanthracene-9,10-dione | |
---|---|---|
Source | PubChem | |
URL | https://pubchem.ncbi.nlm.nih.gov | |
Description | Data deposited in or computed by PubChem | |
InChI |
InChI=1S/C33H40O19/c1-10-5-12-19(24(41)18-11(20(12)37)3-2-4-13(18)36)14(6-10)48-32-27(44)26(43)22(39)17(51-32)9-47-31-29(46)30(23(40)16(8-35)49-31)52-33-28(45)25(42)21(38)15(7-34)50-33/h2-6,15-17,21-23,25-36,38-40,42-46H,7-9H2,1H3 | |
Source | PubChem | |
URL | https://pubchem.ncbi.nlm.nih.gov | |
Description | Data deposited in or computed by PubChem | |
InChI Key |
SOWISUOFXLRAML-UHFFFAOYSA-N | |
Source | PubChem | |
URL | https://pubchem.ncbi.nlm.nih.gov | |
Description | Data deposited in or computed by PubChem | |
Canonical SMILES |
CC1=CC2=C(C(=C1)OC3C(C(C(C(O3)COC4C(C(C(C(O4)CO)O)OC5C(C(C(C(O5)CO)O)O)O)O)O)O)O)C(=O)C6=C(C2=O)C=CC=C6O | |
Source | PubChem | |
URL | https://pubchem.ncbi.nlm.nih.gov | |
Description | Data deposited in or computed by PubChem | |
Molecular Formula |
C33H40O19 | |
Source | PubChem | |
URL | https://pubchem.ncbi.nlm.nih.gov | |
Description | Data deposited in or computed by PubChem | |
Molecular Weight |
740.7 g/mol | |
Source | PubChem | |
URL | https://pubchem.ncbi.nlm.nih.gov | |
Description | Data deposited in or computed by PubChem | |
Physical Description |
Solid | |
Record name | Chrysophanol 1-triglucoside | |
Source | Human Metabolome Database (HMDB) | |
URL | http://www.hmdb.ca/metabolites/HMDB0034571 | |
Description | The Human Metabolome Database (HMDB) is a freely available electronic database containing detailed information about small molecule metabolites found in the human body. | |
Explanation | HMDB is offered to the public as a freely available resource. Use and re-distribution of the data, in whole or in part, for commercial purposes requires explicit permission of the authors and explicit acknowledgment of the source material (HMDB) and the original publication (see the HMDB citing page). We ask that users who download significant portions of the database cite the HMDB paper in any resulting publications. | |
CAS No. |
120181-07-9 | |
Record name | Chrysophanol 1-triglucoside | |
Source | Human Metabolome Database (HMDB) | |
URL | http://www.hmdb.ca/metabolites/HMDB0034571 | |
Description | The Human Metabolome Database (HMDB) is a freely available electronic database containing detailed information about small molecule metabolites found in the human body. | |
Explanation | HMDB is offered to the public as a freely available resource. Use and re-distribution of the data, in whole or in part, for commercial purposes requires explicit permission of the authors and explicit acknowledgment of the source material (HMDB) and the original publication (see the HMDB citing page). We ask that users who download significant portions of the database cite the HMDB paper in any resulting publications. | |
Melting Point |
284 - 286 °C | |
Record name | Chrysophanol 1-triglucoside | |
Source | Human Metabolome Database (HMDB) | |
URL | http://www.hmdb.ca/metabolites/HMDB0034571 | |
Description | The Human Metabolome Database (HMDB) is a freely available electronic database containing detailed information about small molecule metabolites found in the human body. | |
Explanation | HMDB is offered to the public as a freely available resource. Use and re-distribution of the data, in whole or in part, for commercial purposes requires explicit permission of the authors and explicit acknowledgment of the source material (HMDB) and the original publication (see the HMDB citing page). We ask that users who download significant portions of the database cite the HMDB paper in any resulting publications. | |
Disclaimer and Information on In-Vitro Research Products
Please be aware that all articles and product information presented on BenchChem are intended solely for informational purposes. The products available for purchase on BenchChem are specifically designed for in-vitro studies, which are conducted outside of living organisms. In-vitro studies, derived from the Latin term "in glass," involve experiments performed in controlled laboratory settings using cells or tissues. It is important to note that these products are not categorized as medicines or drugs, and they have not received approval from the FDA for the prevention, treatment, or cure of any medical condition, ailment, or disease. We must emphasize that any form of bodily introduction of these products into humans or animals is strictly prohibited by law. It is essential to adhere to these guidelines to ensure compliance with legal and ethical standards in research and experimentation.