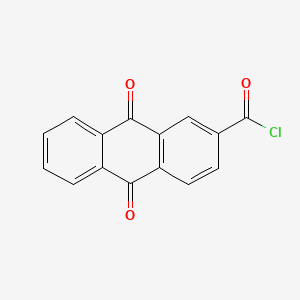
Anthraquinone-2-carbonyl Chloride
Overview
Description
Anthraquinone-2-carbonyl chloride is a derivative of anthraquinone, a compound known for its diverse applications in various fields such as dye manufacturing, medicine, and organic electronics. The compound is characterized by the presence of a carbonyl chloride group attached to the anthraquinone structure, making it a versatile intermediate in organic synthesis .
Mechanism of Action
Target of Action
Anthraquinone-2-carbonyl Chloride, also known as 9,10-Dioxo-9,10-dihydroanthracene-2-carbonyl chloride, has been reported to have several targets of action. It has been used in the synthesis of a polymeric photosensitizer, which is applied in the aerobic photooxidative hydroxylation of boronic acids . Additionally, anthraquinones, a group to which this compound belongs, have been identified as anticancer agents, targeting essential cellular proteins .
Mode of Action
The interaction of this compound with its targets results in significant changes. For instance, in the context of its application in the photooxidative hydroxylation of boronic acids, it plays a crucial role in the synthesis of a polymeric photosensitizer . In the realm of anticancer activity, anthraquinone-based compounds, including this compound, inhibit cancer progression by interacting with essential cellular proteins .
Biochemical Pathways
This compound affects several biochemical pathways. In the process of aerobic photooxidative hydroxylation of boronic acids, it contributes to the synthesis of a polymeric photosensitizer . Furthermore, as an anticancer agent, it impacts key target proteins such as kinases, topoisomerases, telomerases, matrix metalloproteinases, and G-quadruplexes involved in the viability of cancer cells .
Pharmacokinetics
The pharmacokinetics of this compound, like other anthraquinones, involves absorption mainly in the intestines and distribution in blood flow-rich tissues and organs. The metabolic pathways of anthraquinones include hydrolysis, glycuronidation, sulfation, methylation/demethylation, hydroxylation/dehydroxylation, oxidation/reduction (hydrogenation), acetylation, and esterification . The main excretion routes for anthraquinones are the kidney, recta, and gallbladder .
Result of Action
The molecular and cellular effects of this compound’s action are significant. As part of a polymeric photosensitizer, it facilitates the photooxidative hydroxylation of thioethers, yielding sulfoxides highly chemoselectively . In the context of anticancer activity, anthraquinones inhibit cancer cell proliferation, invasion, migration, metastasis, induce cellular apoptosis, and regulate the host immune response .
Action Environment
The action, efficacy, and stability of this compound can be influenced by environmental factors. For instance, the visible light-induced photooxidation of thioethers under aerobic conditions is a key environmental factor in its role as a photosensitizer . In the context of anticancer activity, drug-drug interactions influencing pharmacokinetics may provide insights into drug compatibility .
Biochemical Analysis
Biochemical Properties
Anthraquinone-2-carbonyl Chloride plays a crucial role in biochemical reactions due to its reactive carbonyl chloride group. This group allows the compound to interact with various enzymes, proteins, and other biomolecules. For instance, it can react with alcohols to form esters, which are important intermediates in organic synthesis . Additionally, this compound has been used as a derivatization reagent for alcohols in mass spectrometry, enhancing the detection sensitivity of the analytes . The compound’s ability to form covalent bonds with biomolecules makes it a valuable tool in biochemical research.
Cellular Effects
This compound exhibits significant effects on various types of cells and cellular processes. It has been shown to influence cell function by modulating cell signaling pathways, gene expression, and cellular metabolism. For example, anthraquinone derivatives, including this compound, have been reported to inhibit cancer cell proliferation, induce apoptosis, and regulate the host immune response . These effects are mediated through interactions with key cellular proteins and enzymes, highlighting the compound’s potential as an anticancer agent.
Molecular Mechanism
The molecular mechanism of action of this compound involves its ability to form covalent bonds with biomolecules, leading to enzyme inhibition or activation and changes in gene expression. The compound’s reactive carbonyl chloride group can interact with nucleophilic sites on proteins and enzymes, resulting in the formation of stable adducts . This interaction can inhibit the activity of target enzymes, thereby modulating various biochemical pathways. Additionally, this compound can induce oxidative stress by generating reactive oxygen species (ROS), further influencing cellular processes .
Temporal Effects in Laboratory Settings
In laboratory settings, the effects of this compound can change over time due to its stability and degradation properties. The compound is known to be moisture-sensitive and should be stored under inert gas to maintain its stability . Over time, exposure to moisture and air can lead to the hydrolysis of the carbonyl chloride group, reducing the compound’s reactivity. Long-term studies have shown that this compound can have sustained effects on cellular function, including prolonged inhibition of enzyme activity and persistent changes in gene expression .
Dosage Effects in Animal Models
The effects of this compound vary with different dosages in animal models. At low doses, the compound has been shown to exhibit beneficial effects, such as antioxidant and anticancer activities . At high doses, it can cause toxic or adverse effects, including oxidative stress and cellular damage . Threshold effects have been observed, where the compound’s activity significantly changes at specific dosage levels. These findings underscore the importance of careful dosage optimization in therapeutic applications.
Metabolic Pathways
This compound is involved in various metabolic pathways, including hydrolysis, glycuronidation, and sulfation . These pathways are mediated by liver metabolic enzymes and intestinal flora, leading to the formation of metabolites that can be further processed or excreted. The compound’s interactions with enzymes such as octaketide synthase and other metabolic enzymes play a crucial role in its biotransformation and biological activity .
Transport and Distribution
Within cells and tissues, this compound is transported and distributed through interactions with specific transporters and binding proteins. The compound’s lipophilic nature allows it to diffuse across cell membranes, while its interactions with binding proteins can influence its localization and accumulation . These properties are essential for its bioavailability and efficacy in biochemical applications.
Subcellular Localization
This compound exhibits specific subcellular localization, which can affect its activity and function. The compound can be directed to specific cellular compartments or organelles through targeting signals or post-translational modifications . For example, its incorporation into polymeric photosensitizers has been shown to enhance its localization and activity in photooxidative reactions . Understanding the subcellular distribution of this compound is crucial for optimizing its use in biochemical research and therapeutic applications.
Preparation Methods
Synthetic Routes and Reaction Conditions: Anthraquinone-2-carbonyl chloride can be synthesized through the reaction of anthraquinone with thionyl chloride or oxalyl chloride. The reaction typically occurs under reflux conditions, where the anthraquinone is dissolved in an appropriate solvent such as dichloromethane, and the chlorinating agent is added slowly. The reaction mixture is then heated to facilitate the formation of the carbonyl chloride derivative .
Industrial Production Methods: In industrial settings, the production of this compound often involves the use of large-scale reactors where the reaction conditions are carefully controlled to ensure high yield and purity. The process may include steps such as purification through recrystallization or distillation to remove any impurities .
Types of Reactions:
Substitution Reactions: this compound undergoes nucleophilic substitution reactions where the chloride group is replaced by various nucleophiles such as amines, alcohols, or thiols.
Solvolysis: The compound can undergo solvolysis in various solvents, leading to the formation of anthraquinone derivatives.
Common Reagents and Conditions:
Nucleophiles: Amines, alcohols, thiols.
Solvents: Dichloromethane, ethanol, water.
Conditions: Reflux, presence of a base such as triethylamine.
Major Products:
Amides: Formed from the reaction with amines.
Esters: Formed from the reaction with alcohols.
Thioesters: Formed from the reaction with thiols.
Scientific Research Applications
Comparison with Similar Compounds
Anthraquinone-2-sulfonyl chloride: Similar in structure but contains a sulfonyl chloride group instead of a carbonyl chloride group.
Anthraquinone-2-carboxylic acid: Contains a carboxylic acid group and is used in the synthesis of various anthraquinone derivatives.
Uniqueness: Anthraquinone-2-carbonyl chloride is unique due to its high reactivity and versatility in forming a wide range of derivatives through substitution reactions. Its ability to act as a redox catalyst further enhances its applicability in various fields, making it a valuable compound in both research and industrial applications .
Properties
IUPAC Name |
9,10-dioxoanthracene-2-carbonyl chloride | |
---|---|---|
Source | PubChem | |
URL | https://pubchem.ncbi.nlm.nih.gov | |
Description | Data deposited in or computed by PubChem | |
InChI |
InChI=1S/C15H7ClO3/c16-15(19)8-5-6-11-12(7-8)14(18)10-4-2-1-3-9(10)13(11)17/h1-7H | |
Source | PubChem | |
URL | https://pubchem.ncbi.nlm.nih.gov | |
Description | Data deposited in or computed by PubChem | |
InChI Key |
DGJNWQJOASAMHY-UHFFFAOYSA-N | |
Source | PubChem | |
URL | https://pubchem.ncbi.nlm.nih.gov | |
Description | Data deposited in or computed by PubChem | |
Canonical SMILES |
C1=CC=C2C(=C1)C(=O)C3=C(C2=O)C=C(C=C3)C(=O)Cl | |
Source | PubChem | |
URL | https://pubchem.ncbi.nlm.nih.gov | |
Description | Data deposited in or computed by PubChem | |
Molecular Formula |
C15H7ClO3 | |
Source | PubChem | |
URL | https://pubchem.ncbi.nlm.nih.gov | |
Description | Data deposited in or computed by PubChem | |
DSSTOX Substance ID |
DTXSID10567134 | |
Record name | 9,10-Dioxo-9,10-dihydroanthracene-2-carbonyl chloride | |
Source | EPA DSSTox | |
URL | https://comptox.epa.gov/dashboard/DTXSID10567134 | |
Description | DSSTox provides a high quality public chemistry resource for supporting improved predictive toxicology. | |
Molecular Weight |
270.66 g/mol | |
Source | PubChem | |
URL | https://pubchem.ncbi.nlm.nih.gov | |
Description | Data deposited in or computed by PubChem | |
CAS No. |
6470-87-7 | |
Record name | 9,10-Dioxo-9,10-dihydroanthracene-2-carbonyl chloride | |
Source | EPA DSSTox | |
URL | https://comptox.epa.gov/dashboard/DTXSID10567134 | |
Description | DSSTox provides a high quality public chemistry resource for supporting improved predictive toxicology. | |
Record name | Anthraquinone-2-carbonyl Chloride | |
Source | European Chemicals Agency (ECHA) | |
URL | https://echa.europa.eu/information-on-chemicals | |
Description | The European Chemicals Agency (ECHA) is an agency of the European Union which is the driving force among regulatory authorities in implementing the EU's groundbreaking chemicals legislation for the benefit of human health and the environment as well as for innovation and competitiveness. | |
Explanation | Use of the information, documents and data from the ECHA website is subject to the terms and conditions of this Legal Notice, and subject to other binding limitations provided for under applicable law, the information, documents and data made available on the ECHA website may be reproduced, distributed and/or used, totally or in part, for non-commercial purposes provided that ECHA is acknowledged as the source: "Source: European Chemicals Agency, http://echa.europa.eu/". Such acknowledgement must be included in each copy of the material. ECHA permits and encourages organisations and individuals to create links to the ECHA website under the following cumulative conditions: Links can only be made to webpages that provide a link to the Legal Notice page. | |
Synthesis routes and methods I
Procedure details
Synthesis routes and methods II
Procedure details
Synthesis routes and methods III
Procedure details
Retrosynthesis Analysis
AI-Powered Synthesis Planning: Our tool employs the Template_relevance Pistachio, Template_relevance Bkms_metabolic, Template_relevance Pistachio_ringbreaker, Template_relevance Reaxys, Template_relevance Reaxys_biocatalysis model, leveraging a vast database of chemical reactions to predict feasible synthetic routes.
One-Step Synthesis Focus: Specifically designed for one-step synthesis, it provides concise and direct routes for your target compounds, streamlining the synthesis process.
Accurate Predictions: Utilizing the extensive PISTACHIO, BKMS_METABOLIC, PISTACHIO_RINGBREAKER, REAXYS, REAXYS_BIOCATALYSIS database, our tool offers high-accuracy predictions, reflecting the latest in chemical research and data.
Strategy Settings
Precursor scoring | Relevance Heuristic |
---|---|
Min. plausibility | 0.01 |
Model | Template_relevance |
Template Set | Pistachio/Bkms_metabolic/Pistachio_ringbreaker/Reaxys/Reaxys_biocatalysis |
Top-N result to add to graph | 6 |
Feasible Synthetic Routes
Disclaimer and Information on In-Vitro Research Products
Please be aware that all articles and product information presented on BenchChem are intended solely for informational purposes. The products available for purchase on BenchChem are specifically designed for in-vitro studies, which are conducted outside of living organisms. In-vitro studies, derived from the Latin term "in glass," involve experiments performed in controlled laboratory settings using cells or tissues. It is important to note that these products are not categorized as medicines or drugs, and they have not received approval from the FDA for the prevention, treatment, or cure of any medical condition, ailment, or disease. We must emphasize that any form of bodily introduction of these products into humans or animals is strictly prohibited by law. It is essential to adhere to these guidelines to ensure compliance with legal and ethical standards in research and experimentation.