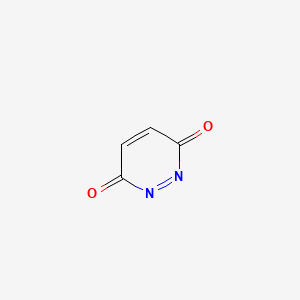
Pyridazine-3,6-dione
Overview
Description
Pyridazine-3,6-dione is a heterocyclic compound with a six-membered ring structure containing two nitrogen atoms . It is isomeric with two other diazine rings, pyrimidine and pyrazine . The molecular weight of this compound is 112.08676 g/mol .
Synthesis Analysis
Pyridazine synthesis involves a Lewis acid-mediated inverse electron demand Diels-Alder reaction between 3-monosubstituted s-tetrazine and silyl enol ethers . An unexpected C-C bond cleavage in the absence of metal enables an efficient approach toward 3,6-diarylpyridazines and 6-arylpyridazin-3-ones from simple and commercially available 1,3-dicarbonyl compounds and methyl ketones .Molecular Structure Analysis
The molecular structure of this compound contains a total of 12 bonds. There are 8 non-H bonds, 3 multiple bonds, 3 double bonds, 1 six-membered ring, and 1 N hydrazine . The 3D chemical structure image of this compound is based on the ball-and-stick model which displays both the three-dimensional position of the atoms and the bonds between them .Chemical Reactions Analysis
This compound has been used in various fields of organic synthesis. It has been involved in [3 + n] cycloaddition reactions, which are one of the most important and efficient tools in the synthesis of heterocyclic rings .Physical And Chemical Properties Analysis
This compound is a colorless liquid with a boiling point of 208 °C . It has a high dipole moment that subtends π-π stacking interactions and robust, dual hydrogen-bonding capacity .Scientific Research Applications
Electrochemical Behavior
- Electrochemical Study in Different Solvents : Pyridazine-3,6-dione shows unique electrochemical behavior, undergoing oxidative ring cleavage to form maleic acid. This process varies depending on the electrolysis media, indicating its potential in electrochemical applications (Varmaghani & Nematollahi, 2011).
Synthesis and Material Applications
- Electrochromic Materials : Pyridazine derivatives have been developed as electron acceptors in electrochromic materials, showcasing properties like good optical contrast and fast switching speed (Ye et al., 2014).
- Chemiluminogens Synthesis : Compounds containing pyridazine units have been synthesized for their chemiluminescent properties, demonstrating potential in various applications, including biosensing (Algi et al., 2017).
Antimicrobial Activity
- Anti-candida Properties : Certain pyridazine derivatives exhibit significant anti-candida activities, opening pathways for medical and pharmaceutical research (Khan, 2018).
Methodological Advances in Synthesis
- Novel Synthesis Methods : Innovative methods for synthesizing pyridazine derivatives have been developed, such as one-pot synthesis techniques and microwave-assisted synthesis, enhancing the efficiency of producing these compounds (Zhang et al., 2011).
Application in Corrosion Inhibition
- Corrosion Inhibitors : Pyridazine derivatives have shown effectiveness as corrosion inhibitors on mild steel, suggesting their utility in industrial applications (Filali et al., 2019).
Pharmaceutical and Biological Potential
- Anticonvulsant Activity : Some pyridazine derivatives have been evaluated as potential anticonvulsant agents, indicating their potential in pharmaceutical research (Sivakumar et al., 2003).
Mechanism of Action
Target of Action
Pyridazine-3,6-dione, also known as 3,6-pyridazinedione, is a heterocyclic compound that contains two adjacent nitrogen atoms. This compound has been shown to have a wide range of pharmacological activities It has been reported to possess anti-inflammatory, anti-fouling, and anti-convulsant properties , suggesting that it may target enzymes or receptors involved in these biological processes.
Mode of Action
For instance, some derivatives of this compound have been found to inhibit prostaglandin E2 and interleukin activity , which could explain its anti-inflammatory properties.
Biochemical Pathways
This compound may affect several biochemical pathways due to its broad spectrum of pharmacological activities. For instance, by inhibiting prostaglandin E2 and interleukin activity, this compound could potentially affect the inflammatory response pathway . .
Result of Action
The molecular and cellular effects of this compound’s action are likely to be diverse, given its wide range of pharmacological activities. For instance, its anti-inflammatory properties could result from the inhibition of prostaglandin E2 and interleukin activity, leading to a reduction in inflammation . .
Safety and Hazards
Future Directions
Pyridazine-3,6-dione has potential applications in medicinal chemistry and optoelectronics . It has been used as a privileged structural element in drug design . The recent approvals of the gonadotropin-releasing hormone receptor antagonist relugolix and the allosteric tyrosine kinase 2 inhibitor deucravacitinib represent the first examples of FDA-approved drugs that incorporate a pyridazine ring .
Biochemical Analysis
Biochemical Properties
Pyridazine-3,6-dione plays a crucial role in several biochemical reactions. It interacts with various enzymes, proteins, and other biomolecules, influencing their activity and function. For instance, this compound has been shown to interact with cyclooxygenase enzymes, inhibiting their activity and thereby reducing the production of pro-inflammatory mediators . Additionally, this compound can form covalent bonds with cysteine residues in proteins, leading to site-selective modification of these proteins . This interaction is particularly useful in the development of targeted therapies and diagnostic tools.
Cellular Effects
This compound exerts significant effects on various types of cells and cellular processes. It has been observed to influence cell signaling pathways, gene expression, and cellular metabolism. For example, this compound can modulate the activity of transcription factors, leading to changes in the expression of genes involved in inflammation and cell proliferation . Furthermore, this compound affects cellular metabolism by inhibiting key metabolic enzymes, resulting in altered metabolic flux and energy production . These effects highlight the potential of this compound as a therapeutic agent for various diseases.
Molecular Mechanism
The mechanism of action of this compound involves several molecular interactions. This compound binds to specific biomolecules, such as enzymes and receptors, altering their activity and function. For instance, this compound inhibits cyclooxygenase enzymes by binding to their active sites, preventing the conversion of arachidonic acid to prostaglandins . Additionally, this compound can form covalent bonds with cysteine residues in proteins, leading to irreversible modification and inhibition of these proteins . These molecular interactions underpin the diverse biological activities of this compound.
Temporal Effects in Laboratory Settings
In laboratory settings, the effects of this compound can change over time due to its stability and degradation. This compound is relatively stable under physiological conditions, but it can undergo hydrolysis and oxidation, leading to the formation of degradation products . These degradation products may have different biological activities compared to the parent compound. Long-term studies have shown that this compound can exert sustained effects on cellular function, including prolonged inhibition of enzyme activity and modulation of gene expression . These findings underscore the importance of considering the temporal dynamics of this compound in experimental settings.
Dosage Effects in Animal Models
The effects of this compound vary with different dosages in animal models. At low doses, this compound has been shown to exert beneficial effects, such as anti-inflammatory and anti-proliferative activities . At high doses, this compound can cause toxic effects, including hepatotoxicity and nephrotoxicity . These adverse effects are likely due to the accumulation of this compound and its metabolites in tissues, leading to cellular damage and dysfunction. Therefore, careful dose optimization is essential to maximize the therapeutic potential of this compound while minimizing its toxicity.
Metabolic Pathways
This compound is involved in several metabolic pathways, interacting with various enzymes and cofactors. It is primarily metabolized by cytochrome P450 enzymes, which catalyze its oxidation and reduction . These metabolic reactions lead to the formation of reactive intermediates that can further interact with cellular macromolecules, such as proteins and nucleic acids . This compound also affects metabolic flux by inhibiting key enzymes in glycolysis and the tricarboxylic acid cycle, resulting in altered levels of metabolites and energy production . These metabolic effects contribute to the overall biological activity of this compound.
Transport and Distribution
This compound is transported and distributed within cells and tissues through various mechanisms. It can diffuse across cell membranes due to its relatively small size and lipophilicity . Additionally, this compound can be actively transported by specific transporters, such as organic anion transporters and multidrug resistance proteins . These transporters facilitate the uptake and efflux of this compound, influencing its intracellular concentration and localization. The distribution of this compound within tissues is also affected by its binding to plasma proteins, which can modulate its bioavailability and pharmacokinetics .
Subcellular Localization
The subcellular localization of this compound is critical for its activity and function. This compound can localize to specific cellular compartments, such as the cytoplasm, nucleus, and mitochondria . This localization is influenced by targeting signals and post-translational modifications that direct this compound to specific organelles . For example, this compound can be targeted to the mitochondria through the action of mitochondrial targeting sequences, where it can modulate mitochondrial function and energy production . The subcellular localization of this compound is essential for its role in regulating cellular processes and responses.
properties
IUPAC Name |
pyridazine-3,6-dione | |
---|---|---|
Source | PubChem | |
URL | https://pubchem.ncbi.nlm.nih.gov | |
Description | Data deposited in or computed by PubChem | |
InChI |
InChI=1S/C4H2N2O2/c7-3-1-2-4(8)6-5-3/h1-2H | |
Source | PubChem | |
URL | https://pubchem.ncbi.nlm.nih.gov | |
Description | Data deposited in or computed by PubChem | |
InChI Key |
ISPBSPVVFOBQEQ-UHFFFAOYSA-N | |
Source | PubChem | |
URL | https://pubchem.ncbi.nlm.nih.gov | |
Description | Data deposited in or computed by PubChem | |
Canonical SMILES |
C1=CC(=O)N=NC1=O | |
Source | PubChem | |
URL | https://pubchem.ncbi.nlm.nih.gov | |
Description | Data deposited in or computed by PubChem | |
Molecular Formula |
C4H2N2O2 | |
Source | PubChem | |
URL | https://pubchem.ncbi.nlm.nih.gov | |
Description | Data deposited in or computed by PubChem | |
DSSTOX Substance ID |
DTXSID30514988 | |
Record name | Pyridazine-3,6-dione | |
Source | EPA DSSTox | |
URL | https://comptox.epa.gov/dashboard/DTXSID30514988 | |
Description | DSSTox provides a high quality public chemistry resource for supporting improved predictive toxicology. | |
Molecular Weight |
110.07 g/mol | |
Source | PubChem | |
URL | https://pubchem.ncbi.nlm.nih.gov | |
Description | Data deposited in or computed by PubChem | |
CAS RN |
42413-70-7 | |
Record name | Pyridazine-3,6-dione | |
Source | EPA DSSTox | |
URL | https://comptox.epa.gov/dashboard/DTXSID30514988 | |
Description | DSSTox provides a high quality public chemistry resource for supporting improved predictive toxicology. | |
Retrosynthesis Analysis
AI-Powered Synthesis Planning: Our tool employs the Template_relevance Pistachio, Template_relevance Bkms_metabolic, Template_relevance Pistachio_ringbreaker, Template_relevance Reaxys, Template_relevance Reaxys_biocatalysis model, leveraging a vast database of chemical reactions to predict feasible synthetic routes.
One-Step Synthesis Focus: Specifically designed for one-step synthesis, it provides concise and direct routes for your target compounds, streamlining the synthesis process.
Accurate Predictions: Utilizing the extensive PISTACHIO, BKMS_METABOLIC, PISTACHIO_RINGBREAKER, REAXYS, REAXYS_BIOCATALYSIS database, our tool offers high-accuracy predictions, reflecting the latest in chemical research and data.
Strategy Settings
Precursor scoring | Relevance Heuristic |
---|---|
Min. plausibility | 0.01 |
Model | Template_relevance |
Template Set | Pistachio/Bkms_metabolic/Pistachio_ringbreaker/Reaxys/Reaxys_biocatalysis |
Top-N result to add to graph | 6 |
Feasible Synthetic Routes
Q & A
Q1: What is the molecular formula and weight of 3,6-pyridazinedione?
A1: The molecular formula of 3,6-pyridazinedione is C4H4N2O2 and its molecular weight is 112.09 g/mol.
Q2: What spectroscopic data is available for characterizing 3,6-pyridazinedione?
A2: Researchers have used various spectroscopic techniques to characterize 3,6-pyridazinedione and its derivatives. These techniques include:
- Mass Spectrometry (MS): Confirms the molecular weight and provides information about fragmentation patterns. []
Q3: Why is the planar configuration of 3,6-pyridazinedione unstable?
A3: The instability of planar 3,6-pyridazinedione is attributed to the pseudo Jahn–Teller effect (PJTE). This effect describes the vibronic coupling between the ground electronic state (1A1) and the first excited state (1A2) along the a2 distortion coordinate, leading to a distortion from the planar configuration to a more stable twisted geometry with C2 symmetry. [] This twisting instability is also observed in 1,2-dihalo derivatives of 3,6-pyridazinedione. []
Q4: What are some common methods for synthesizing 3,6-pyridazinedione derivatives?
A4: Several synthetic routes have been developed for 3,6-pyridazinedione derivatives:
- Reaction of maleic anhydride with hydrazine: This method is commonly used to synthesize 1,2-dihydro-3,6-pyridazinedione, also known as maleic hydrazide. [, ]
- Electrochemical reduction of azo compounds: This approach utilizes electrochemical methods to synthesize tetrahydro-1,2-substituted 3-pyridazinones and 3,6-pyridazinediones. [, ]
- Cycloaddition reactions: Researchers have explored cycloaddition reactions of pyridazine-3,6-diones with various dienophiles to create diverse derivatives. [, ]
- Condensation reactions: N-amino-N,N'-dihydrodiazinediones can undergo condensation reactions with 1,3-dicarbonyl compounds or α,β-unsaturated carbonyl compounds to produce fused nitrogen-heterocycles. [, ]
Q5: How does Lawesson's reagent react with 3,6-pyridazinedione?
A5: The reaction of Lawesson's reagent with 3,6-pyridazinedione derivatives can lead to the formation of various products depending on the specific reaction conditions and the substituents present on the pyridazinedione ring. These products include:
- Dithiones: Lawesson's reagent can convert the carbonyl groups of 3,6-pyridazinedione into thiocarbonyl groups, resulting in dithione derivatives. []
- Monothiones: Depending on the reaction conditions and stoichiometry, monothione derivatives can also be formed, where only one of the carbonyl groups is converted to a thiocarbonyl group. []
- Disulfides: In some cases, the reaction can lead to the formation of disulfide derivatives, particularly when using 1-phenyl-1,2-dihydro-3,6-pyridazinedione as the starting material. []
- 1,3,2-Oxathiophosphole derivatives: These heterocyclic compounds can be obtained as byproducts in certain reactions of 1-phenyl-1,2-dihydro-3,6-pyridazinedione with Lawesson's reagent. []
Q6: What are the primary applications of 3,6-pyridazinedione and its derivatives?
A6: 3,6-pyridazinedione derivatives have found applications in various fields:
- Agriculture: Maleic hydrazide (1,2-dihydro-3,6-pyridazinedione) is a commercially available plant growth regulator used to inhibit sprout growth in stored potatoes and onions. [, ] It's also been investigated for its potential to control weeds, suppress seedhead formation in grasses, and improve forage quality. [, , , , ]
- Medicinal chemistry: Some derivatives exhibit cytotoxic activity and have been investigated as potential anticancer agents. [, , ] Researchers have explored their activity against various cancer cell lines. [, , ]
- Organic synthesis: 3,6-pyridazinedione serves as a versatile building block for synthesizing diverse nitrogen-containing heterocyclic compounds. []
Q7: What is the mechanism of action of maleic hydrazide as a plant growth regulator?
A7: While the exact mechanism of action of maleic hydrazide is not fully elucidated, it is believed to interfere with cell division and elongation by disrupting DNA synthesis. [] This inhibition of cell division contributes to its sprout suppression activity in stored potatoes and onions. [, ]
Q8: How does the structure of 3,6-pyridazinedione derivatives relate to their biological activity?
A8: Structure-activity relationship (SAR) studies have shown that modifications to the 3,6-pyridazinedione scaffold can significantly impact biological activity.
- Halogenation: Introduction of halogens, particularly bromine, at positions 4 and 5 of the pyridazine ring has been associated with increased cytotoxic activity in some derivatives. [, ] This effect is thought to be related to both the electronic properties and the lipophilicity of the halogen substituents. [, ]
- Substitution at nitrogen atoms: N-substitution with various groups can influence the compound's interaction with biological targets and modulate its activity. [] For example, the anticonvulsant activity of 1-substituted-1,2-dihydro-pyridazine-3,6-diones has been investigated, with varying degrees of protection against seizures depending on the substituent. []
Q9: Are there any known resistance mechanisms to 3,6-pyridazinedione derivatives?
A9: While resistance mechanisms specific to 3,6-pyridazinedione derivatives have not been extensively studied, the prolonged use of maleic hydrazide in agriculture could potentially lead to the development of resistant weed populations. Monitoring for resistance development and implementing appropriate resistance management strategies, such as crop rotation and the use of alternative weed control methods, are crucial to minimize the risk of resistance.
Q10: What is known about the metabolism of maleic hydrazide in plants?
A10: Research has identified a novel metabolite of maleic hydrazide in tobacco plants: MH-N-β-D-glucoside. [] This finding suggests that plants can metabolize maleic hydrazide by forming glycosidic conjugates. Further research is needed to fully understand the metabolic pathways of maleic hydrazide in different plant species and the potential implications for its efficacy and environmental fate.
Q11: What is the environmental fate of maleic hydrazide?
A11: Maleic hydrazide can persist in the environment, particularly in soil, for extended periods. [] Factors influencing its degradation include soil type, pH, temperature, and microbial activity. [] Research on the photocatalytic degradation of maleic hydrazide using titanium dioxide (TiO2) suggests a potential strategy for removing this herbicide derivative from water sources. []
- Computational chemistry: Computational studies can provide valuable insights into the molecular properties, reactivity, and interactions of 3,6-pyridazinedione derivatives with biological targets. These studies can guide the design of novel derivatives with improved activity, selectivity, and pharmacokinetic properties. [, ]
- Drug delivery and targeting: Developing targeted drug delivery systems for 3,6-pyridazinedione derivatives could enhance their therapeutic efficacy while minimizing potential side effects. []
- Biomarkers and diagnostics: Research on biomarkers associated with the activity of 3,6-pyridazinedione derivatives could contribute to personalized medicine approaches for optimizing treatment outcomes. []
Disclaimer and Information on In-Vitro Research Products
Please be aware that all articles and product information presented on BenchChem are intended solely for informational purposes. The products available for purchase on BenchChem are specifically designed for in-vitro studies, which are conducted outside of living organisms. In-vitro studies, derived from the Latin term "in glass," involve experiments performed in controlled laboratory settings using cells or tissues. It is important to note that these products are not categorized as medicines or drugs, and they have not received approval from the FDA for the prevention, treatment, or cure of any medical condition, ailment, or disease. We must emphasize that any form of bodily introduction of these products into humans or animals is strictly prohibited by law. It is essential to adhere to these guidelines to ensure compliance with legal and ethical standards in research and experimentation.