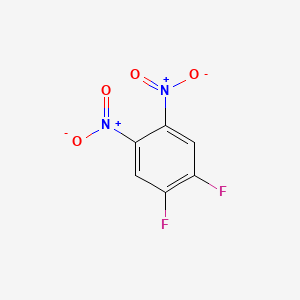
1,2-Difluoro-4,5-dinitrobenzene
Overview
Description
1,2-Difluoro-4,5-dinitrobenzene is a useful research compound. Its molecular formula is C6H2F2N2O4 and its molecular weight is 204.09 g/mol. The purity is usually 95%.
BenchChem offers high-quality this compound suitable for many research applications. Different packaging options are available to accommodate customers' requirements. Please inquire for more information about this compound including the price, delivery time, and more detailed information at info@benchchem.com.
Mechanism of Action
Target of Action
It’s known that this compound can interact with various amines .
Mode of Action
It’s known that the compound can undergo reactions with amines . The fluorine atoms in the compound are always displaced in preference to the nitro groups .
Biochemical Pathways
It’s known that the compound can participate in various organic synthesis reactions .
Action Environment
The action, efficacy, and stability of 1,2-Difluoro-4,5-dinitrobenzene can be influenced by environmental factors. For instance, the compound is soluble in some organic solvents such as ether and dimethyl sulfoxide . This solubility can influence its action and efficacy in different environments.
Biochemical Analysis
Biochemical Properties
1,2-Difluoro-4,5-dinitrobenzene plays a crucial role in various biochemical reactions. It is known to interact with a range of enzymes, proteins, and other biomolecules. One of the primary interactions involves the modification of amino groups in proteins, which can lead to changes in protein function and activity. For instance, this compound has been shown to react with amino acids such as alanine, aspartic acid, glutamic acid, methionine, and phenylalanine, forming stable derivatives that can be analyzed using high-performance liquid chromatography . These interactions are primarily driven by the reactive fluorine atoms and nitro groups, which facilitate the formation of covalent bonds with amino groups.
Cellular Effects
The effects of this compound on various types of cells and cellular processes are profound. This compound can influence cell function by altering cell signaling pathways, gene expression, and cellular metabolism. For example, this compound has been observed to inhibit the activity of certain enzymes involved in metabolic pathways, leading to changes in the levels of key metabolites . Additionally, it can affect the expression of genes related to stress response and detoxification, thereby impacting cellular homeostasis and function.
Molecular Mechanism
At the molecular level, this compound exerts its effects through several mechanisms. One of the primary mechanisms involves the covalent modification of proteins and enzymes, which can result in enzyme inhibition or activation. The reactive fluorine atoms in this compound can form covalent bonds with nucleophilic amino acid residues, such as lysine and cysteine, leading to changes in enzyme activity . Additionally, the nitro groups can participate in redox reactions, further influencing the biochemical properties of the compound. These molecular interactions can lead to alterations in gene expression, as the modified proteins may act as transcription factors or signaling molecules.
Temporal Effects in Laboratory Settings
The effects of this compound can change over time in laboratory settings. This compound is relatively stable under standard laboratory conditions, but it can undergo degradation over extended periods or under specific conditions, such as exposure to light or heat . Long-term studies have shown that this compound can have lasting effects on cellular function, including persistent changes in enzyme activity and gene expression. These temporal effects are important to consider when designing experiments and interpreting results.
Dosage Effects in Animal Models
The effects of this compound vary with different dosages in animal models. At low doses, this compound may have minimal impact on cellular function, while higher doses can lead to significant changes in metabolism and gene expression . Threshold effects have been observed, where a certain dosage is required to elicit a measurable response. Additionally, high doses of this compound can be toxic, leading to adverse effects such as oxidative stress and cellular damage. These dosage-dependent effects are critical for understanding the safety and efficacy of this compound in biological systems.
Properties
IUPAC Name |
1,2-difluoro-4,5-dinitrobenzene | |
---|---|---|
Source | PubChem | |
URL | https://pubchem.ncbi.nlm.nih.gov | |
Description | Data deposited in or computed by PubChem | |
InChI |
InChI=1S/C6H2F2N2O4/c7-3-1-5(9(11)12)6(10(13)14)2-4(3)8/h1-2H | |
Source | PubChem | |
URL | https://pubchem.ncbi.nlm.nih.gov | |
Description | Data deposited in or computed by PubChem | |
InChI Key |
XOAAAPHKZDETSB-UHFFFAOYSA-N | |
Source | PubChem | |
URL | https://pubchem.ncbi.nlm.nih.gov | |
Description | Data deposited in or computed by PubChem | |
Canonical SMILES |
C1=C(C(=CC(=C1F)F)[N+](=O)[O-])[N+](=O)[O-] | |
Source | PubChem | |
URL | https://pubchem.ncbi.nlm.nih.gov | |
Description | Data deposited in or computed by PubChem | |
Molecular Formula |
C6H2F2N2O4 | |
Source | PubChem | |
URL | https://pubchem.ncbi.nlm.nih.gov | |
Description | Data deposited in or computed by PubChem | |
DSSTOX Substance ID |
DTXSID10507358 | |
Record name | 1,2-Difluoro-4,5-dinitrobenzene | |
Source | EPA DSSTox | |
URL | https://comptox.epa.gov/dashboard/DTXSID10507358 | |
Description | DSSTox provides a high quality public chemistry resource for supporting improved predictive toxicology. | |
Molecular Weight |
204.09 g/mol | |
Source | PubChem | |
URL | https://pubchem.ncbi.nlm.nih.gov | |
Description | Data deposited in or computed by PubChem | |
CAS No. |
85686-97-1 | |
Record name | 1,2-Difluoro-4,5-dinitrobenzene | |
Source | EPA DSSTox | |
URL | https://comptox.epa.gov/dashboard/DTXSID10507358 | |
Description | DSSTox provides a high quality public chemistry resource for supporting improved predictive toxicology. | |
Record name | 1,2-difluoro-4,5-dinitrobenzene | |
Source | European Chemicals Agency (ECHA) | |
URL | https://echa.europa.eu/information-on-chemicals | |
Description | The European Chemicals Agency (ECHA) is an agency of the European Union which is the driving force among regulatory authorities in implementing the EU's groundbreaking chemicals legislation for the benefit of human health and the environment as well as for innovation and competitiveness. | |
Explanation | Use of the information, documents and data from the ECHA website is subject to the terms and conditions of this Legal Notice, and subject to other binding limitations provided for under applicable law, the information, documents and data made available on the ECHA website may be reproduced, distributed and/or used, totally or in part, for non-commercial purposes provided that ECHA is acknowledged as the source: "Source: European Chemicals Agency, http://echa.europa.eu/". Such acknowledgement must be included in each copy of the material. ECHA permits and encourages organisations and individuals to create links to the ECHA website under the following cumulative conditions: Links can only be made to webpages that provide a link to the Legal Notice page. | |
Retrosynthesis Analysis
AI-Powered Synthesis Planning: Our tool employs the Template_relevance Pistachio, Template_relevance Bkms_metabolic, Template_relevance Pistachio_ringbreaker, Template_relevance Reaxys, Template_relevance Reaxys_biocatalysis model, leveraging a vast database of chemical reactions to predict feasible synthetic routes.
One-Step Synthesis Focus: Specifically designed for one-step synthesis, it provides concise and direct routes for your target compounds, streamlining the synthesis process.
Accurate Predictions: Utilizing the extensive PISTACHIO, BKMS_METABOLIC, PISTACHIO_RINGBREAKER, REAXYS, REAXYS_BIOCATALYSIS database, our tool offers high-accuracy predictions, reflecting the latest in chemical research and data.
Strategy Settings
Precursor scoring | Relevance Heuristic |
---|---|
Min. plausibility | 0.01 |
Model | Template_relevance |
Template Set | Pistachio/Bkms_metabolic/Pistachio_ringbreaker/Reaxys/Reaxys_biocatalysis |
Top-N result to add to graph | 6 |
Feasible Synthetic Routes
Q1: What makes 1,2-difluoro-4,5-dinitrobenzene particularly reactive?
A1: The presence of both fluorine atoms and nitro groups on the benzene ring makes this compound highly reactive. [, ] Specifically, the fluorine atoms are preferentially substituted over the nitro groups in reactions with nucleophiles like amines. [] This selectivity is likely due to the high electronegativity of fluorine, making the carbon atoms to which they are attached more susceptible to nucleophilic attack.
Q2: How has this compound been used in the synthesis of new compounds?
A2: Researchers have successfully utilized this compound as a starting material to synthesize novel phenoxazines and phenothiazines. [] For instance, reacting this compound with 2-aminophenol or 2-(N-methylamino)phenol yields the corresponding 2,3-dinitrophenoxazines. These newly synthesized compounds can be further modified by replacing the nitro group conjugated to the aryl ether with various nucleophiles like butylamine, potassium ethoxide, or potassium hydroxide. []
Q3: Are there any potential applications of this compound beyond organic synthesis?
A3: Yes, research suggests that this compound holds promise as an insensitive melt-castable energetic material. [] This application is likely attributed to the presence of both the electron-withdrawing nitro groups and the energetic C-F bonds.
Q4: Where can I find additional information regarding the structural characterization of this compound and its derivatives?
A4: Several studies utilize X-ray single crystal structure determinations to characterize this compound and the compounds derived from its reactions with various nucleophiles. [] These analyses provide valuable insights into the dihedral angles, bond lengths, and overall molecular geometry of these molecules.
Disclaimer and Information on In-Vitro Research Products
Please be aware that all articles and product information presented on BenchChem are intended solely for informational purposes. The products available for purchase on BenchChem are specifically designed for in-vitro studies, which are conducted outside of living organisms. In-vitro studies, derived from the Latin term "in glass," involve experiments performed in controlled laboratory settings using cells or tissues. It is important to note that these products are not categorized as medicines or drugs, and they have not received approval from the FDA for the prevention, treatment, or cure of any medical condition, ailment, or disease. We must emphasize that any form of bodily introduction of these products into humans or animals is strictly prohibited by law. It is essential to adhere to these guidelines to ensure compliance with legal and ethical standards in research and experimentation.