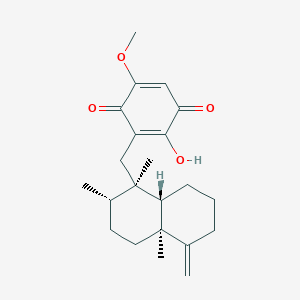
Ilimaquinone
Overview
Description
Ilimaquinone is a sesquiterpene quinone compound that was first isolated from the marine sponge Smenospongia cerebriformis. It is known for its unique chemical structure, which includes a benzoquinone moiety combined with a trans-decalin ring system bearing an exocyclic double bond . This compound has garnered significant interest due to its diverse biological activities and potential therapeutic applications.
Mechanism of Action
Target of Action
Ilimaquinone (IQ) is a metabolite found in marine sponges . It has been reported to have a number of biological properties, including potential anticancer activity . The primary targets of IQ include Pyruvate Dehydrogenase Kinase 1 (PDK1) and several key proteins of SARS-CoV-2 . PDK1 plays a crucial role in energy metabolism, and its inhibition can shift the energy metabolism from aerobic glycolysis to oxidative phosphorylation . The SARS-CoV-2 proteins are vital for viral replication .
Mode of Action
IQ interacts with its targets, leading to significant changes in their function. For instance, IQ inhibits PDK1, reducing the phosphorylation of its substrate, Pyruvate Dehydrogenase (PDH) . This shifts the energy metabolism from aerobic glycolysis to oxidative phosphorylation . IQ also inhibits several key proteins of SARS-CoV-2, potentially hindering viral replication .
Biochemical Pathways
IQ affects several biochemical pathways. By inhibiting PDK1, it impacts the energy metabolism pathway , shifting it from aerobic glycolysis to oxidative phosphorylation . This can lead to the generation of mitochondrial reactive oxygen species (ROS) and depolarization of the mitochondrial membrane potential . IQ also affects the apoptosis pathway . It induces apoptosis in cancer cells via the mitochondrial-mediated apoptosis pathway .
Pharmacokinetics
The pharmacokinetics of IQ and its epimer, epi-IQ, have been studied in rats . Both substances decay in a bi-exponential pattern, with an elimination rate constant of 1.2 h−1 for IQ and 1.7 h−1 for epi-IQ . Epi-IQ is distributed more widely than IQ by about two-fold, and consequently, the clearance of epi-IQ is greater than that of IQ by about three-fold . The oral absolute bioavailability for IQ is 38%, and that for epi-IQ is 13% .
Result of Action
IQ has significant effects at the molecular and cellular levels. It suppresses the viability of cancer cells in a dose-dependent manner . It induces apoptotic cell death, as evidenced by fluorescent microscopy, flow cytometry, DNA fragmentation, and the TUNEL assay . It also increases the level of caspase-3 and caspase-9 expression and downregulates Bcl-2 .
Action Environment
The action of IQ can be influenced by environmental factors. For instance, the marine environment, where IQ is naturally found, could potentially affect its action, efficacy, and stability . .
Biochemical Analysis
Biochemical Properties
Ilimaquinone interacts with various enzymes and proteins, notably pyruvate dehydrogenase kinase 1 (PDK1) . It inhibits the activity of PDK1, which plays a crucial role in energy metabolism .
Cellular Effects
This compound has been shown to decrease the viability of human and murine cancer cells, such as A549, DLD-1, RKO, and LLC cells . It influences cell function by shifting energy metabolism from aerobic glycolysis to oxidative phosphorylation .
Molecular Mechanism
This compound exerts its effects at the molecular level by binding to PDK1 and inhibiting its activity . This leads to a decrease in the phosphorylation of pyruvate dehydrogenase, the substrate of PDK1 . As a result, it shifts the energy metabolism from aerobic glycolysis to oxidative phosphorylation .
Temporal Effects in Laboratory Settings
It has been shown to decrease lactate production and increase oxygen consumption .
Metabolic Pathways
This compound is involved in the metabolic pathway of energy production. It interacts with PDK1, an enzyme that regulates the activity of pyruvate dehydrogenase, a key enzyme in the pathway of oxidative phosphorylation .
Preparation Methods
Synthetic Routes and Reaction Conditions: The synthesis of ilimaquinone involves several steps, including the formation of the quinone moiety and the construction of the trans-decalin ring system. One common synthetic route involves the radical decarboxylation and quinone addition reaction . The reaction conditions typically require the use of reagents such as lithium enolate and benzyl halide, followed by exposure to light and maintaining the temperature at 0-5°C .
Industrial Production Methods: Industrial production of this compound is not widely established due to its complex structure and the challenges associated with its synthesis. advancements in synthetic organic chemistry may pave the way for more efficient production methods in the future.
Chemical Reactions Analysis
Types of Reactions: Ilimaquinone undergoes various chemical reactions, including:
Oxidation: this compound can be oxidized to form different quinone derivatives.
Reduction: Reduction of this compound can lead to the formation of hydroquinone derivatives.
Substitution: this compound can undergo substitution reactions, particularly at the quinone moiety.
Common Reagents and Conditions:
Oxidation: Common oxidizing agents include potassium permanganate and chromium trioxide.
Reduction: Reducing agents such as sodium borohydride and lithium aluminum hydride are often used.
Substitution: Substitution reactions may involve reagents like halogens and nucleophiles under acidic or basic conditions.
Major Products Formed: The major products formed from these reactions include various quinone and hydroquinone derivatives, which may exhibit different biological activities.
Scientific Research Applications
Chemistry: Ilimaquinone serves as a valuable compound for studying quinone chemistry and developing new synthetic methodologies.
Medicine: this compound exhibits anticancer properties and has been investigated for its potential use in cancer therapy.
Industry: While industrial applications are limited, this compound’s unique structure and biological activities make it a compound of interest for future pharmaceutical developments.
Comparison with Similar Compounds
Ilimaquinone can be compared with other sesquiterpene quinones and related compounds:
Similar Compounds: Radicicol, M77976, and other resorcinol-containing compounds share structural similarities with this compound.
Uniqueness: this compound’s unique combination of a benzoquinone moiety and trans-decalin ring system, along with its diverse biological activities, sets it apart from other similar compounds.
Properties
IUPAC Name |
3-[[(1R,2S,4aS,8aS)-1,2,4a-trimethyl-5-methylidene-3,4,6,7,8,8a-hexahydro-2H-naphthalen-1-yl]methyl]-2-hydroxy-5-methoxycyclohexa-2,5-diene-1,4-dione | |
---|---|---|
Source | PubChem | |
URL | https://pubchem.ncbi.nlm.nih.gov | |
Description | Data deposited in or computed by PubChem | |
InChI |
InChI=1S/C22H30O4/c1-13-7-6-8-18-21(13,3)10-9-14(2)22(18,4)12-15-19(24)16(23)11-17(26-5)20(15)25/h11,14,18,24H,1,6-10,12H2,2-5H3/t14-,18+,21+,22+/m0/s1 | |
Source | PubChem | |
URL | https://pubchem.ncbi.nlm.nih.gov | |
Description | Data deposited in or computed by PubChem | |
InChI Key |
JJWITJNSXCXULM-YVUMSICPSA-N | |
Source | PubChem | |
URL | https://pubchem.ncbi.nlm.nih.gov | |
Description | Data deposited in or computed by PubChem | |
Canonical SMILES |
CC1CCC2(C(C1(C)CC3=C(C(=O)C=C(C3=O)OC)O)CCCC2=C)C | |
Source | PubChem | |
URL | https://pubchem.ncbi.nlm.nih.gov | |
Description | Data deposited in or computed by PubChem | |
Isomeric SMILES |
C[C@H]1CC[C@]2([C@H]([C@]1(C)CC3=C(C(=O)C=C(C3=O)OC)O)CCCC2=C)C | |
Source | PubChem | |
URL | https://pubchem.ncbi.nlm.nih.gov | |
Description | Data deposited in or computed by PubChem | |
Molecular Formula |
C22H30O4 | |
Source | PubChem | |
URL | https://pubchem.ncbi.nlm.nih.gov | |
Description | Data deposited in or computed by PubChem | |
DSSTOX Substance ID |
DTXSID90221909 | |
Record name | Illimaquinone | |
Source | EPA DSSTox | |
URL | https://comptox.epa.gov/dashboard/DTXSID90221909 | |
Description | DSSTox provides a high quality public chemistry resource for supporting improved predictive toxicology. | |
Molecular Weight |
358.5 g/mol | |
Source | PubChem | |
URL | https://pubchem.ncbi.nlm.nih.gov | |
Description | Data deposited in or computed by PubChem | |
CAS No. |
71678-03-0 | |
Record name | Ilimaquinone | |
Source | CAS Common Chemistry | |
URL | https://commonchemistry.cas.org/detail?cas_rn=71678-03-0 | |
Description | CAS Common Chemistry is an open community resource for accessing chemical information. Nearly 500,000 chemical substances from CAS REGISTRY cover areas of community interest, including common and frequently regulated chemicals, and those relevant to high school and undergraduate chemistry classes. This chemical information, curated by our expert scientists, is provided in alignment with our mission as a division of the American Chemical Society. | |
Explanation | The data from CAS Common Chemistry is provided under a CC-BY-NC 4.0 license, unless otherwise stated. | |
Record name | Imaquinone | |
Source | ChemIDplus | |
URL | https://pubchem.ncbi.nlm.nih.gov/substance/?source=chemidplus&sourceid=0071678030 | |
Description | ChemIDplus is a free, web search system that provides access to the structure and nomenclature authority files used for the identification of chemical substances cited in National Library of Medicine (NLM) databases, including the TOXNET system. | |
Record name | Illimaquinone | |
Source | EPA DSSTox | |
URL | https://comptox.epa.gov/dashboard/DTXSID90221909 | |
Description | DSSTox provides a high quality public chemistry resource for supporting improved predictive toxicology. | |
Record name | ILIMAQUINONE | |
Source | FDA Global Substance Registration System (GSRS) | |
URL | https://gsrs.ncats.nih.gov/ginas/app/beta/substances/5U0WAN3N8Q | |
Description | The FDA Global Substance Registration System (GSRS) enables the efficient and accurate exchange of information on what substances are in regulated products. Instead of relying on names, which vary across regulatory domains, countries, and regions, the GSRS knowledge base makes it possible for substances to be defined by standardized, scientific descriptions. | |
Explanation | Unless otherwise noted, the contents of the FDA website (www.fda.gov), both text and graphics, are not copyrighted. They are in the public domain and may be republished, reprinted and otherwise used freely by anyone without the need to obtain permission from FDA. Credit to the U.S. Food and Drug Administration as the source is appreciated but not required. | |
Retrosynthesis Analysis
AI-Powered Synthesis Planning: Our tool employs the Template_relevance Pistachio, Template_relevance Bkms_metabolic, Template_relevance Pistachio_ringbreaker, Template_relevance Reaxys, Template_relevance Reaxys_biocatalysis model, leveraging a vast database of chemical reactions to predict feasible synthetic routes.
One-Step Synthesis Focus: Specifically designed for one-step synthesis, it provides concise and direct routes for your target compounds, streamlining the synthesis process.
Accurate Predictions: Utilizing the extensive PISTACHIO, BKMS_METABOLIC, PISTACHIO_RINGBREAKER, REAXYS, REAXYS_BIOCATALYSIS database, our tool offers high-accuracy predictions, reflecting the latest in chemical research and data.
Strategy Settings
Precursor scoring | Relevance Heuristic |
---|---|
Min. plausibility | 0.01 |
Model | Template_relevance |
Template Set | Pistachio/Bkms_metabolic/Pistachio_ringbreaker/Reaxys/Reaxys_biocatalysis |
Top-N result to add to graph | 6 |
Feasible Synthetic Routes
Q1: How does ilimaquinone exert its cytotoxic effects?
A1: this compound's cytotoxicity is attributed to several mechanisms:
- DNA Damage: this compound, specifically its active hydroquinone form, can directly cleave DNA. [] In cellular environments, both this compound and its precursors can damage cellular DNA, likely through in situ conversion to hydroquinones. []
- Apoptosis Induction: this compound triggers apoptosis through various pathways, including mitochondrial dysfunction, caspase activation (caspase-3 and -9), and modulation of Bcl-2 family proteins. [, ]
- Autophagy Induction: this compound can induce autophagy, a cellular degradation process, which may play a protective role against its cytotoxic effects. [, ]
- Inhibition of Pyruvate Dehydrogenase Kinase 1 (PDK1): this compound inhibits PDK1, leading to a shift in energy metabolism from aerobic glycolysis to oxidative phosphorylation. This metabolic shift contributes to its anticancer activity. []
Q2: What is the role of the hydroquinone form of this compound in its activity?
A2: While this compound itself exhibits biological activity, research suggests that its hydroquinone form is the active species responsible for DNA cleavage. [] This conversion likely occurs within the cellular environment, explaining the activity of both this compound and its precursors in cell-based assays. []
Q3: Does this compound interact with specific proteins?
A3: Yes, this compound has been shown to interact with several proteins:* β-catenin: this compound inhibits Wnt/β-catenin signaling by downregulating β-catenin levels, leading to the suppression of multiple myeloma cell proliferation. []* p53: this compound activates the p53 pathway, leading to p53 stabilization, upregulation of p21WAF1/CIP1 expression, and the induction of apoptosis and autophagy in colon cancer cells. []
Q4: What is the molecular formula and weight of this compound?
A4: The molecular formula of this compound is C17H22O3, and its molecular weight is 274.36 g/mol. []
Q5: Are there spectroscopic data available for this compound?
A5: Yes, spectroscopic data, including NMR (Nuclear Magnetic Resonance), IR (Infrared), and Mass spectrometry, are widely available for this compound and its derivatives. These data are crucial for structural elucidation and are reported in numerous research articles. [, , , , , ]
Q6: How do structural modifications of this compound impact its biological activity?
A6: Structural modifications significantly influence this compound's activity:* Hydroxyl Group at C-2: The presence of a hydroxyl group at the C-2 position of the quinone moiety is crucial for inhibiting Pyruvate Phosphate Dikinase (PPDK) activity. []* C-20 Side Chain: A short hydroxide/alkoxide side chain at C-20 favors PPDK inhibition, while a larger amine side chain is better tolerated for phospholipase A2 (PLA2) inhibition. []* Stereochemistry: The two epimers, this compound and 5-epi-ilimaquinone, exhibit differences in cytotoxicity and apoptosis induction, indicating the importance of stereochemistry for biological activity. []
Q7: What is known about the stability of this compound?
A7: this compound's stability is influenced by factors such as pH and the presence of reducing agents. [] The epimer 5-epi-ilimaquinone generally exhibits higher stability than this compound in rat plasma. []
Q8: What is the pharmacokinetic profile of this compound?
A8: this compound and its epimer, 5-epi-ilimaquinone, display stereo-selective pharmacokinetics in rats. [] Epi-ilimaquinone shows higher stability in plasma, wider distribution, and greater clearance compared to this compound. [] The oral bioavailability of this compound is higher than that of epi-ilimaquinone. []
Q9: What types of in vitro and in vivo studies have been conducted with this compound?
A9: this compound has been extensively studied in various models:
- Cell-based assays: Demonstrated cytotoxicity against multiple human cancer cell lines, including breast cancer [], colon cancer [, ], multiple myeloma [], and oral squamous cell carcinoma. []
- Zebrafish model: Showed potential in attenuating heart failure induced by arachidonic acid (AA) and renal toxins. []
- Starfish oocytes: Exhibited inhibitory effects on oocyte maturation. []
Q10: How is this compound typically analyzed and quantified?
A10: HPLC-MS/MS (High-Performance Liquid Chromatography coupled with tandem Mass Spectrometry) is a commonly employed technique for the simultaneous determination and quantification of this compound and its epimer in biological matrices like plasma. []
Disclaimer and Information on In-Vitro Research Products
Please be aware that all articles and product information presented on BenchChem are intended solely for informational purposes. The products available for purchase on BenchChem are specifically designed for in-vitro studies, which are conducted outside of living organisms. In-vitro studies, derived from the Latin term "in glass," involve experiments performed in controlled laboratory settings using cells or tissues. It is important to note that these products are not categorized as medicines or drugs, and they have not received approval from the FDA for the prevention, treatment, or cure of any medical condition, ailment, or disease. We must emphasize that any form of bodily introduction of these products into humans or animals is strictly prohibited by law. It is essential to adhere to these guidelines to ensure compliance with legal and ethical standards in research and experimentation.