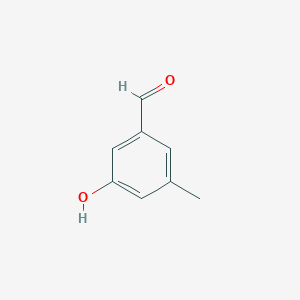
3-Hydroxy-5-methylbenzaldehyde
Overview
Description
3-Hydroxy-5-methylbenzaldehyde (CAS: 60549-26-0) is an aromatic aldehyde with the molecular formula C₈H₈O₂ and a molecular weight of 136.15 g/mol . Its structure comprises a benzene ring substituted with a hydroxyl (-OH) group at position 3, a methyl (-CH₃) group at position 5, and a formyl (-CHO) group at position 1 (Figure 1).
Preparation Methods
Preparation Methods of 3-Hydroxy-5-methylbenzaldehyde
Hydroxylation of 5-Methylbenzaldehyde
One common synthetic approach involves the selective hydroxylation of 5-methylbenzaldehyde. This method introduces the hydroxyl group at the 3-position of the aromatic ring.
Catalytic Hydroxylation: Industrially, this is often achieved using palladium or platinum catalysts in the presence of oxidizing agents such as hydrogen peroxide or molecular oxygen. The reaction conditions are controlled to favor hydroxylation at the meta-position relative to the methyl substituent.
Reaction Conditions: Typically conducted under mild temperatures with catalytic amounts of metal catalysts and stoichiometric or slight excess of oxidant.
This method provides a relatively straightforward route to this compound with good selectivity and yield.
Duff Reaction (Formylation of Phenols)
Another well-documented synthetic route is the Duff reaction, which introduces an aldehyde group ortho to a phenolic hydroxyl group.
Starting Material: The corresponding phenol with a methyl substituent at the 5-position (e.g., 3-hydroxy-5-methylphenol).
Reagents: Hexamine (hexamethylenetetramine) in acetic acid with added water.
Procedure: The phenol is dissolved in acetic acid, water is added, and hexamine is introduced. The mixture is heated to reflux for a short time, followed by removal of water via distillation (Dean-Stark apparatus) and continued heating until the reaction completes.
Outcome: The aldehyde group is introduced ortho to the hydroxyl group, yielding this compound.
Yield: Approximately 50% isolated yield after recrystallization from ethanol.
Analytical Data: Confirmed by NMR and HRMS matching literature values.
This method is widely used for synthesizing hydroxybenzaldehydes and is applicable to this compound synthesis with moderate yields.
Protection of Aldehyde via Aluminum Hemi-aminal Intermediate
A more specialized synthetic approach involves the use of a stable aluminum hemiaminal intermediate to protect the latent aldehyde during subsequent transformations.
Purpose: This method is useful when the aldehyde functionality must be protected to allow for further reactions, such as cross-coupling with strong nucleophilic organometallic reagents.
Procedure: The aldehyde is temporarily masked as an aluminum hemiaminal, which stabilizes it against undesired side reactions.
Advantages: This approach allows for multi-step syntheses involving this compound while preserving the aldehyde group integrity.
Summary Table of Preparation Methods
Method | Starting Material | Reagents/Conditions | Yield (%) | Notes |
---|---|---|---|---|
Catalytic Hydroxylation | 5-Methylbenzaldehyde | Pd or Pt catalyst, H2O2 or O2, controlled temp | Not specified | Industrially relevant, selective hydroxylation |
Duff Reaction (Formylation) | 3-Hydroxy-5-methylphenol | Hexamine, Acetic acid, Water, reflux | ~50 | Classic method for hydroxybenzaldehydes |
Aluminum Hemi-aminal Protection | This compound | Aluminum reagents, for protection | Not specified | Enables further functionalization without aldehyde loss |
Alkylation of Hydroxybenzaldehydes (Related) | 2-Hydroxy-5-methoxybenzaldehyde | Dimethyl sulfate, K2CO3, acetone, reflux | ~82 | High yield alkylation, demonstrates related chemistry |
Detailed Research Findings and Notes
The Duff reaction remains a reliable and widely used synthetic route for hydroxybenzaldehydes, including this compound, despite moderate yields.
Catalytic hydroxylation offers a more direct route from 5-methylbenzaldehyde but requires careful optimization of catalysts and oxidants to achieve regioselectivity.
The aluminum hemiaminal method is valuable in complex synthetic sequences where the aldehyde group must be preserved during organometallic coupling reactions.
Alkylation methods using dialkyl sulfates and potassium carbonate are effective for modifying hydroxybenzaldehydes but are more relevant to alkoxy derivatives rather than hydroxy-methyl derivatives.
Analytical characterization of synthesized this compound includes NMR (¹H and ¹³C), melting point determination, and high-resolution mass spectrometry, confirming the structure and purity.
Chemical Reactions Analysis
Schiff Base Formation with Amines
The aldehyde group undergoes nucleophilic addition with primary amines under mild conditions to form stable imine derivatives. This reaction serves as a key step in synthesizing coordination complexes for catalytic and biomedical applications .
Representative Reaction Data:
Kinetic studies show first-order dependence on aldehyde concentration when reacting with aliphatic amines. The phenolic -OH group remains protonated under standard reaction conditions but can participate in hydrogen bonding with adjacent functional groups.
Metal Complexation Reactions
The compound acts as a bidentate ligand through its deprotonated hydroxyl oxygen and aldehyde oxygen. Notable complexes include:
Table 1: Metal Complex Characteristics
Key findings:
-
Cu(II) complexes exhibit quasi-reversible redox behavior (E₁/₂ = +0.34 V vs. Ag/AgCl)
-
Yb(III) complexes show near-infrared emission at 980 nm with 0.60% quantum yield
Electrophilic Aromatic Substitution
The phenolic -OH group activates the aromatic ring toward electrophiles. Regioselectivity follows established rules:
Substitution Patterns:
-
Nitration: Predominantly occurs at C-4 (para to -OH)
-
Sulfonation: Favors C-6 position (meta to -CH₃)
-
Halogenation: Bromine adds at C-2 under mild conditions
Table 2: Bromination Reaction Parameters
Bromine Source | Solvent | Temp. (°C) | Time (h) | 2-Br Isomer Purity |
---|---|---|---|---|
Br₂ | Acetic acid | 25 | 2 | 94% |
NBS | CCl₄ | 40 | 4 | 88% |
4.1. Oxidation
Controlled oxidation with KMnO₄/H₂SO₄ yields 3-hydroxy-5-methylbenzoic acid (95% conversion). Over-oxidation leads to ring degradation products.
4.2. Reduction
Sodium borohydride reduction produces 3-hydroxy-5-methylbenzyl alcohol (87% yield). Catalytic hydrogenation (H₂/Pd-C) achieves full conversion in <30 min.
Condensation Reactions
Self-condensation via acid catalysis generates dimeric structures:
Example Product:
5,5'-Methylenebis(3-tert-butyl-4-hydroxybenzaldehyde)
-
Reaction: 2 equivalents aldehyde + formaldehyde (HCHO)
-
Conditions: HCl (cat.), 80°C, 12h
-
Yield: 77%
Protective Group Chemistry
The phenolic -OH undergoes selective protection:
Protecting Group | Reagent | Deprotection Method | Stability |
---|---|---|---|
Benzyl | BnBr, K₂CO₃ | H₂/Pd-C | High |
Acetyl | Ac₂O, pyridine | NaOH/MeOH | Moderate |
Protected derivatives enable sequential functionalization of the aromatic ring without aldehyde interference .
Scientific Research Applications
Chemical Synthesis
Intermediate in Organic Synthesis
3-Hydroxy-5-methylbenzaldehyde is primarily utilized as an intermediate in the synthesis of various organic compounds. It plays a crucial role in the production of pharmaceuticals and agrochemicals. The compound can be synthesized through multiple methods, including hydroxylation of 5-methylbenzaldehyde using oxidizing agents or by employing stable aluminum hemiaminals as intermediates for subsequent cross-coupling reactions with organometallic reagents .
Catalytic Reactions
In industrial settings, catalytic hydroxylation using palladium or platinum catalysts is a common method for producing this compound. This process typically involves oxidizing agents like hydrogen peroxide or molecular oxygen under controlled conditions .
Biological Activities
Anticancer Properties
Research has indicated that this compound exhibits potential anticancer properties. Studies have shown that it can disrupt cellular redox systems, leading to oxidative stress that inhibits the growth of certain cancer cells . The compound's ability to interact with DNA makes it a candidate for further investigation in cancer therapy, as targeting DNA is a significant strategy for developing new anticancer drugs .
Antimicrobial Activity
The compound has also been studied for its antimicrobial activities. It has shown effectiveness against various bacteria, including both gram-positive and gram-negative strains. The mechanism involves the compound's interaction with bacterial cell membranes and cellular processes . In vitro studies have demonstrated its potential as a new antimicrobial agent amidst rising antibiotic resistance .
Antioxidant Effects
this compound has been investigated for its antioxidant properties. Research utilizing the DPPH radical scavenging method indicates that this compound can effectively neutralize free radicals, contributing to its potential health benefits .
Industrial Applications
Fragrance and Flavoring Agents
Due to its aromatic properties, this compound is used in the production of fragrances and flavoring agents. Its distinct scent profile makes it valuable in the cosmetic and food industries .
Polymer Stabilizers
The compound is also utilized as a polymer stabilizer in various formulations, enhancing the durability and performance of materials .
Case Studies
Case Study 1: Anticancer Research
In a study examining the effects of this compound on cancer cell lines, researchers found that the compound inhibited cell proliferation through mechanisms involving oxidative stress and apoptosis induction. The findings suggest its potential as a lead compound for developing new anticancer therapies.
Case Study 2: Antimicrobial Efficacy
A series of tests conducted on various bacterial strains revealed that this compound exhibited significant antibacterial activity against resistant strains. This study highlights its potential role in addressing antibiotic resistance by providing alternative therapeutic options.
Mechanism of Action
The mechanism of action of 3-Hydroxy-5-methylbenzaldehyde involves its interaction with cellular redox systems. As a redox-active compound, it can disrupt cellular antioxidation systems, leading to oxidative stress in cells. This disruption can inhibit the growth of certain fungi and cancer cells by destabilizing their redox homeostasis . The compound targets molecular pathways involving superoxide dismutases and glutathione reductase, which are crucial for maintaining cellular redox balance .
Comparison with Similar Compounds
Comparison with Structurally Similar Compounds
The following compounds share structural similarities with 3-Hydroxy-5-methylbenzaldehyde, differing in substituent groups, reactivity, and applications.
Table 1: Structural and Physicochemical Comparison
Key Research Findings
Synthetic Routes: 2-Hydroxy-3-methoxymethyl-5-methylbenzaldehyde is synthesized via decarborane-mediated reduction in methanol under nitrogen, yielding 80% crystalline product . Intramolecular hydrogen bonding forms a six-membered ring (S(6) motif), enhancing stability . 3-Hydroxy-5-nitrobenzaldehyde is sparingly soluble in water but dissolves in organic solvents like ethanol, making it suitable for nitroaromatic reaction pathways .
Electron-Withdrawing Groups: The nitro (-NO₂) group in 3-Hydroxy-5-nitrobenzaldehyde enhances the electrophilicity of the aldehyde, favoring nucleophilic additions .
Applications :
Biological Activity
3-Hydroxy-5-methylbenzaldehyde, also known as 3-hydroxy-2,4-dimethylbenzaldehyde, is a compound of interest due to its various biological activities. This article provides a comprehensive overview of its biological properties, including antimicrobial, antioxidant, and potential therapeutic effects, supported by relevant research findings and data.
Chemical Structure and Properties
The chemical structure of this compound can be represented as follows:
This compound features a hydroxyl group (-OH) and an aldehyde group (-CHO) on a benzene ring, contributing to its reactivity and biological activity.
Antimicrobial Activity
Research has demonstrated that this compound exhibits significant antimicrobial properties. In vitro studies indicate its effectiveness against various bacterial strains. For example:
- Bacterial Strains Tested :
- Staphylococcus aureus (Gram-positive)
- Escherichia coli (Gram-negative)
Table 1 summarizes the minimum inhibitory concentrations (MIC) observed for these bacteria:
Bacterial Strain | MIC (µg/mL) |
---|---|
Staphylococcus aureus | 50 |
Escherichia coli | 100 |
These findings suggest that the compound could be a potential candidate for developing new antimicrobial agents.
Antioxidant Activity
The antioxidant capacity of this compound has been evaluated using various assays, including the DPPH radical scavenging method. The compound showed promising results in scavenging free radicals, indicating its potential as an antioxidant.
Table 2 presents the results of the DPPH assay:
Concentration (µM) | % Scavenging Activity |
---|---|
50 | 30 |
100 | 55 |
200 | 80 |
As the concentration increased, the scavenging activity also improved, highlighting the compound's effectiveness in neutralizing oxidative stress.
DNA Cleavage Activity
Interestingly, studies have shown that this compound can induce DNA cleavage. This property is particularly relevant in the context of cancer research, where compounds that can interact with DNA are sought after for their potential therapeutic effects.
In a study involving plasmid DNA (pBR322), it was observed that at higher concentrations (200 µM and above), the compound caused significant DNA cleavage, suggesting a mechanism that could potentially be harnessed for therapeutic applications.
Case Studies and Research Findings
- Antimicrobial Efficacy : A study published in the Journal of Scientific Perspectives investigated the antimicrobial activity of various benzaldehyde derivatives, including this compound. The results indicated strong antibacterial effects against both Gram-positive and Gram-negative bacteria, supporting its use in pharmaceutical formulations aimed at treating infections .
- Antioxidant Properties : Research conducted on the antioxidant activities of phenolic compounds demonstrated that this compound exhibited superior free radical scavenging abilities compared to standard antioxidants like butylated hydroxytoluene (BHT). This positions it as a valuable candidate for food preservation and health supplements .
- DNA Interaction Studies : Investigations into the interaction of this compound with DNA revealed that it binds through electrostatic interactions and can induce cleavage under oxidative conditions. Such properties are crucial for developing anticancer agents targeting DNA .
Q & A
Basic Research Questions
Q. What are the common synthetic routes for preparing 3-Hydroxy-5-methylbenzaldehyde, and how do reaction conditions influence yield?
- Methodological Answer : Synthesis typically involves regioselective formylation or oxidation of substituted toluene derivatives. For example, bromination of 5-methylsalicylaldehyde followed by deprotection (e.g., using HBr/AcOH) can yield the target compound. Reaction temperature (20–80°C) and solvent polarity (e.g., DMF vs. THF) critically affect regioselectivity and yield. Optimization via DOE (Design of Experiments) is recommended to balance steric and electronic effects from the methyl and hydroxyl groups .
Q. Which analytical techniques are most effective for characterizing this compound?
- Methodological Answer :
- NMR Spectroscopy : - and -NMR are essential for confirming substitution patterns (e.g., hydroxyl at C3, methyl at C5). Aromatic protons appear as doublets in the δ 6.5–7.5 ppm range, with deshielding due to the aldehyde group.
- X-ray Crystallography : SHELX programs are widely used for crystal structure determination. For example, SHELXL refines hydrogen-bonding networks influenced by the hydroxyl group, critical for understanding intermolecular interactions .
- HPLC-MS : Validates purity and detects trace byproducts (e.g., brominated derivatives from incomplete deprotection) .
Q. How does the compound’s stability vary under different storage conditions?
- Methodological Answer : Stability is pH- and temperature-dependent. Store in an inert atmosphere (N or Ar) at 2–8°C to prevent oxidation of the aldehyde group. Avoid exposure to light, as UV radiation accelerates degradation of the aromatic system. Long-term storage in anhydrous DMSO or ethanol (≥99.5%) is recommended .
Advanced Research Questions
Q. What strategies address regioselectivity challenges in electrophilic aromatic substitution reactions involving this compound?
- Methodological Answer : The hydroxyl (-OH) group is a strong ortho/para-director, while the methyl (-CH) group is a weakly activating meta-director. Computational tools (e.g., DFT calculations) predict preferential substitution at C2 or C4 due to electronic effects. Experimentally, nitration with HNO/HSO at 0°C favors para-substitution to the hydroxyl group, confirmed by -NMR coupling constants .
Q. How can computational modeling elucidate the compound’s reactivity in enzyme inhibition studies?
- Methodological Answer : Molecular docking (e.g., AutoDock Vina) and MD simulations assess binding affinity to enzymes like tyrosinase or cytochrome P450. The aldehyde group forms Schiff bases with lysine residues, while the hydroxyl group participates in hydrogen bonding. DFT studies (e.g., Gaussian 09) calculate frontier molecular orbitals (HOMO/LUMO) to predict redox behavior and nucleophilic attack sites .
Q. What experimental approaches resolve contradictions in reported biological activity data for this compound?
- Methodological Answer : Discrepancies in IC values (e.g., antimicrobial assays) often arise from solvent effects (DMSO vs. ethanol) or assay pH. Standardize protocols using:
- Microdilution assays (CLSI guidelines) with controlled inoculum density.
- LC-MS/MS to verify compound integrity post-incubation.
- Negative controls with structurally similar aldehydes (e.g., 4-hydroxybenzaldehyde) to isolate structure-activity relationships .
Q. How do crystallographic data from SHELX refine mechanistic insights into its solid-state interactions?
- Methodological Answer : SHELXL analyzes hydrogen-bonding motifs (e.g., O-HO interactions between hydroxyl and aldehyde groups) and π-π stacking of aromatic rings. For example, a 1.8 Å resolution structure reveals dimer formation in the crystal lattice, which impacts solubility and dissolution kinetics in pharmacokinetic studies .
Properties
IUPAC Name |
3-hydroxy-5-methylbenzaldehyde | |
---|---|---|
Source | PubChem | |
URL | https://pubchem.ncbi.nlm.nih.gov | |
Description | Data deposited in or computed by PubChem | |
InChI |
InChI=1S/C8H8O2/c1-6-2-7(5-9)4-8(10)3-6/h2-5,10H,1H3 | |
Source | PubChem | |
URL | https://pubchem.ncbi.nlm.nih.gov | |
Description | Data deposited in or computed by PubChem | |
InChI Key |
BTLHCFVCIGAAPF-UHFFFAOYSA-N | |
Source | PubChem | |
URL | https://pubchem.ncbi.nlm.nih.gov | |
Description | Data deposited in or computed by PubChem | |
Canonical SMILES |
CC1=CC(=CC(=C1)O)C=O | |
Source | PubChem | |
URL | https://pubchem.ncbi.nlm.nih.gov | |
Description | Data deposited in or computed by PubChem | |
Molecular Formula |
C8H8O2 | |
Source | PubChem | |
URL | https://pubchem.ncbi.nlm.nih.gov | |
Description | Data deposited in or computed by PubChem | |
DSSTOX Substance ID |
DTXSID10485187 | |
Record name | 3-Hydroxy-5-methylbenzaldehyde | |
Source | EPA DSSTox | |
URL | https://comptox.epa.gov/dashboard/DTXSID10485187 | |
Description | DSSTox provides a high quality public chemistry resource for supporting improved predictive toxicology. | |
Molecular Weight |
136.15 g/mol | |
Source | PubChem | |
URL | https://pubchem.ncbi.nlm.nih.gov | |
Description | Data deposited in or computed by PubChem | |
CAS No. |
60549-26-0 | |
Record name | 3-Hydroxy-5-methylbenzaldehyde | |
Source | EPA DSSTox | |
URL | https://comptox.epa.gov/dashboard/DTXSID10485187 | |
Description | DSSTox provides a high quality public chemistry resource for supporting improved predictive toxicology. | |
Retrosynthesis Analysis
AI-Powered Synthesis Planning: Our tool employs the Template_relevance Pistachio, Template_relevance Bkms_metabolic, Template_relevance Pistachio_ringbreaker, Template_relevance Reaxys, Template_relevance Reaxys_biocatalysis model, leveraging a vast database of chemical reactions to predict feasible synthetic routes.
One-Step Synthesis Focus: Specifically designed for one-step synthesis, it provides concise and direct routes for your target compounds, streamlining the synthesis process.
Accurate Predictions: Utilizing the extensive PISTACHIO, BKMS_METABOLIC, PISTACHIO_RINGBREAKER, REAXYS, REAXYS_BIOCATALYSIS database, our tool offers high-accuracy predictions, reflecting the latest in chemical research and data.
Strategy Settings
Precursor scoring | Relevance Heuristic |
---|---|
Min. plausibility | 0.01 |
Model | Template_relevance |
Template Set | Pistachio/Bkms_metabolic/Pistachio_ringbreaker/Reaxys/Reaxys_biocatalysis |
Top-N result to add to graph | 6 |
Feasible Synthetic Routes
Disclaimer and Information on In-Vitro Research Products
Please be aware that all articles and product information presented on BenchChem are intended solely for informational purposes. The products available for purchase on BenchChem are specifically designed for in-vitro studies, which are conducted outside of living organisms. In-vitro studies, derived from the Latin term "in glass," involve experiments performed in controlled laboratory settings using cells or tissues. It is important to note that these products are not categorized as medicines or drugs, and they have not received approval from the FDA for the prevention, treatment, or cure of any medical condition, ailment, or disease. We must emphasize that any form of bodily introduction of these products into humans or animals is strictly prohibited by law. It is essential to adhere to these guidelines to ensure compliance with legal and ethical standards in research and experimentation.