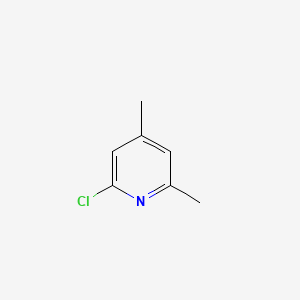
2-Chloro-4,6-dimethylpyridine
Overview
Description
2-Chloro-4,6-dimethylpyridine is an organic compound with the formula C6H7NCl. It is a colorless liquid that is soluble in many organic solvents. It is used in the synthesis of pharmaceuticals, agrochemicals, and other organic compounds. It is also used as a catalyst in certain chemical reactions. It is a versatile reagent and has been used in a variety of scientific research applications.
Scientific Research Applications
Synthesis of 2-anilinopyrimidines
2-Chloro-4,6-dimethylpyrimidine is used in the synthesis of 2-anilinopyrimidines . This process involves aromatic nucleophilic substitution with differently substituted anilines under microwave conditions . The substituents have a significant impact on the course and efficiency of the reaction .
Organic Synthesis
2-Chloro-4,6-dimethylpyridine is an important raw material and intermediate used in organic synthesis . It is used in the production of various organic compounds, contributing to the diversity and complexity of organic chemistry .
Agrochemical Applications
This compound is also used in the agrochemical field . It serves as a key ingredient in the production of certain agrochemicals, contributing to the development of more effective and efficient agricultural practices .
Pharmaceutical Applications
In the pharmaceutical field, 2-Chloro-4,6-dimethylpyridine is used as a raw material and intermediate . It plays a crucial role in the synthesis of various pharmaceutical compounds, contributing to advancements in medical treatments .
Dyestuff Field
2-Chloro-4,6-dimethylpyridine is used in the dyestuff field . It is involved in the production of certain dyes, contributing to the color and quality of various products .
Synthesis of Pyrazol Derivatives
The reaction of 2-chloro-4,6-dimethylpyrimidine-3-carbonitrile with hydrazine hydrate, hydroxylamine, and anthranilic acid affords the corresponding pyrazol . This highlights its role in the synthesis of pyrazol derivatives, which have various applications in medicinal chemistry .
properties
IUPAC Name |
2-chloro-4,6-dimethylpyridine | |
---|---|---|
Source | PubChem | |
URL | https://pubchem.ncbi.nlm.nih.gov | |
Description | Data deposited in or computed by PubChem | |
InChI |
InChI=1S/C7H8ClN/c1-5-3-6(2)9-7(8)4-5/h3-4H,1-2H3 | |
Source | PubChem | |
URL | https://pubchem.ncbi.nlm.nih.gov | |
Description | Data deposited in or computed by PubChem | |
InChI Key |
COVGXNSRDOYAJD-UHFFFAOYSA-N | |
Source | PubChem | |
URL | https://pubchem.ncbi.nlm.nih.gov | |
Description | Data deposited in or computed by PubChem | |
Canonical SMILES |
CC1=CC(=NC(=C1)Cl)C | |
Source | PubChem | |
URL | https://pubchem.ncbi.nlm.nih.gov | |
Description | Data deposited in or computed by PubChem | |
Molecular Formula |
C7H8ClN | |
Source | PubChem | |
URL | https://pubchem.ncbi.nlm.nih.gov | |
Description | Data deposited in or computed by PubChem | |
DSSTOX Substance ID |
DTXSID20500794 | |
Record name | 2-Chloro-4,6-dimethylpyridine | |
Source | EPA DSSTox | |
URL | https://comptox.epa.gov/dashboard/DTXSID20500794 | |
Description | DSSTox provides a high quality public chemistry resource for supporting improved predictive toxicology. | |
Molecular Weight |
141.60 g/mol | |
Source | PubChem | |
URL | https://pubchem.ncbi.nlm.nih.gov | |
Description | Data deposited in or computed by PubChem | |
Product Name |
2-Chloro-4,6-dimethylpyridine | |
CAS RN |
30838-93-8 | |
Record name | 2-Chloro-4,6-dimethylpyridine | |
Source | EPA DSSTox | |
URL | https://comptox.epa.gov/dashboard/DTXSID20500794 | |
Description | DSSTox provides a high quality public chemistry resource for supporting improved predictive toxicology. | |
Record name | 2-Chloro-4,6-dimethylpyridine | |
Source | European Chemicals Agency (ECHA) | |
URL | https://echa.europa.eu/information-on-chemicals | |
Description | The European Chemicals Agency (ECHA) is an agency of the European Union which is the driving force among regulatory authorities in implementing the EU's groundbreaking chemicals legislation for the benefit of human health and the environment as well as for innovation and competitiveness. | |
Explanation | Use of the information, documents and data from the ECHA website is subject to the terms and conditions of this Legal Notice, and subject to other binding limitations provided for under applicable law, the information, documents and data made available on the ECHA website may be reproduced, distributed and/or used, totally or in part, for non-commercial purposes provided that ECHA is acknowledged as the source: "Source: European Chemicals Agency, http://echa.europa.eu/". Such acknowledgement must be included in each copy of the material. ECHA permits and encourages organisations and individuals to create links to the ECHA website under the following cumulative conditions: Links can only be made to webpages that provide a link to the Legal Notice page. | |
Synthesis routes and methods I
Procedure details
Synthesis routes and methods II
Procedure details
Retrosynthesis Analysis
AI-Powered Synthesis Planning: Our tool employs the Template_relevance Pistachio, Template_relevance Bkms_metabolic, Template_relevance Pistachio_ringbreaker, Template_relevance Reaxys, Template_relevance Reaxys_biocatalysis model, leveraging a vast database of chemical reactions to predict feasible synthetic routes.
One-Step Synthesis Focus: Specifically designed for one-step synthesis, it provides concise and direct routes for your target compounds, streamlining the synthesis process.
Accurate Predictions: Utilizing the extensive PISTACHIO, BKMS_METABOLIC, PISTACHIO_RINGBREAKER, REAXYS, REAXYS_BIOCATALYSIS database, our tool offers high-accuracy predictions, reflecting the latest in chemical research and data.
Strategy Settings
Precursor scoring | Relevance Heuristic |
---|---|
Min. plausibility | 0.01 |
Model | Template_relevance |
Template Set | Pistachio/Bkms_metabolic/Pistachio_ringbreaker/Reaxys/Reaxys_biocatalysis |
Top-N result to add to graph | 6 |
Feasible Synthetic Routes
Q & A
Q1: What are the structural characteristics of 2-chloro-4,6-dimethylpyridine and how have these been studied?
A: While the provided abstract mentioning 2-chloro-4,6-dimethylpyridine does not offer specific structural data, the paper focusing on 2-hydroxy-4,6-dimethylpyridine-3-carbonitrile and 2-chloro-4,6-dimethylpyridine-3-carbonitrile offers insights. [] Researchers used Density Functional Theory (DFT) calculations with the B3LYP/6-31G* method to optimize the structures of these compounds in gas and aqueous solutions. [] This computational approach helps determine bond lengths, angles, and overall molecular geometry. Further analysis of vibrational frequencies, atomic charges, and dipole moments contributes to understanding the compound's behavior and potential interactions. []
Q2: How does the presence of aqueous solution impact the properties of 2-chloro-4,6-dimethylpyridine-3-carbonitrile?
A: The study employed the Integral Equation Formalism of the Polarizable Continuum Model (IEFPCM) with the Self Consistent Reaction Field (SCRF) method to simulate the impact of an aqueous environment on the compound. [] This allowed researchers to investigate how solvent interactions influence the compound's structure, charge distribution, and overall stability. These findings provide crucial information for understanding the compound's behavior in biological systems, where water is a primary solvent.
Disclaimer and Information on In-Vitro Research Products
Please be aware that all articles and product information presented on BenchChem are intended solely for informational purposes. The products available for purchase on BenchChem are specifically designed for in-vitro studies, which are conducted outside of living organisms. In-vitro studies, derived from the Latin term "in glass," involve experiments performed in controlled laboratory settings using cells or tissues. It is important to note that these products are not categorized as medicines or drugs, and they have not received approval from the FDA for the prevention, treatment, or cure of any medical condition, ailment, or disease. We must emphasize that any form of bodily introduction of these products into humans or animals is strictly prohibited by law. It is essential to adhere to these guidelines to ensure compliance with legal and ethical standards in research and experimentation.