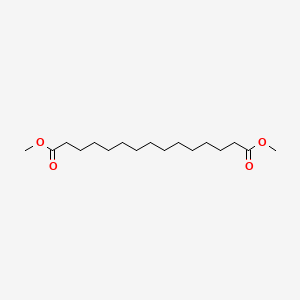
Dimethyl Pentadecanedioate
Overview
Description
Dimethyl pentadecanedioate (CAS No. 36575-82-3), also known as pentadecanedioic acid dimethyl ester, is a diester compound with the molecular formula C₁₇H₃₂O₄ and a molecular weight of 300.43 g/mol . It is primarily used as a laboratory chemical for research and development purposes . Physically, it exists as a solid with a melting point of 43–44°C . Its synthesis from Malania oleifera oil has been optimized, with yields reaching 72.4% under specific esterification conditions .
Preparation Methods
Dimethyl Pentadecanedioate can be synthesized through several methods. One common synthetic route involves the esterification of pentadecanedioic acid with methanol in the presence of an acid catalyst such as sulfuric acid. The reaction typically occurs under reflux conditions, and the product is purified through distillation or recrystallization .
Industrial production methods often involve the use of more efficient catalysts and optimized reaction conditions to increase yield and reduce production costs. For example, the use of solid acid catalysts can enhance the esterification process, making it more suitable for large-scale production .
Chemical Reactions Analysis
Dimethyl Pentadecanedioate undergoes various chemical reactions, including:
Oxidation: It can be oxidized to form pentadecanedioic acid using oxidizing agents such as potassium permanganate or chromium trioxide.
Reduction: Reduction of this compound can yield pentadecanediol when treated with reducing agents like lithium aluminum hydride.
Common reagents and conditions used in these reactions include acidic or basic catalysts, varying temperatures, and solvents like ethanol or dichloromethane. The major products formed from these reactions depend on the specific reagents and conditions used .
Scientific Research Applications
Applications in Polymer Chemistry
DMPD is primarily utilized in the synthesis of polyesters and polyurethanes. Its long carbon chain provides flexibility and enhances the mechanical properties of the resulting polymers.
Polyester Production
- Application : DMPD serves as a monomer in the production of high-performance polyesters.
- Case Study : Research has shown that incorporating DMPD into polyester formulations results in materials with improved thermal stability and mechanical strength compared to traditional polyesters derived from shorter-chain diacids.
Polyurethane Synthesis
- Application : DMPD is used in the production of polyurethanes, particularly in coatings and adhesives.
- Case Study : A study demonstrated that polyurethanes synthesized with DMPD exhibited enhanced abrasion resistance and flexibility, making them suitable for automotive and industrial applications.
Applications in Specialty Chemicals
DMPD is also employed as a precursor in the synthesis of various specialty chemicals, including plasticizers, surfactants, and lubricants.
Plasticizers
- Application : DMPD is used to produce plasticizers that improve the flexibility and durability of plastics.
- Data Table :
Plasticizer Type | Composition | Properties |
---|---|---|
Phthalate-free | DMPD-based | Low volatility, high compatibility |
Traditional | Phthalates | Higher volatility, potential health risks |
Surfactants
- Application : DMPD can be modified to create surfactants used in detergents and emulsifiers.
- Case Study : A formulation study indicated that surfactants derived from DMPD showed superior emulsifying properties compared to those derived from shorter-chain esters.
Biological Applications
Emerging research suggests potential applications of DMPD in biomedical fields, particularly as a scaffold material for drug delivery systems due to its biocompatibility.
Drug Delivery Systems
- Application : DMPD-based polymers can be designed for controlled release of therapeutic agents.
- Case Study : Experimental results revealed that drug-loaded DMPD-based nanoparticles exhibited sustained release profiles suitable for long-term therapies.
Mechanism of Action
The mechanism by which Dimethyl Pentadecanedioate exerts its effects varies depending on its application. In chemical reactions, it typically acts as a substrate that undergoes transformation through catalytic processes. In biological systems, it may interact with enzymes that catalyze the hydrolysis of ester bonds, leading to the formation of pentadecanedioic acid and methanol .
Comparison with Similar Compounds
Dimethyl pentadecanedioate belongs to a family of linear aliphatic diesters. Below is a comparative analysis with structurally analogous compounds:
Molecular and Physical Properties
Key Observations :
- Chain Length and Physical State : this compound’s solid state contrasts with dimethyl hexadecanedioate’s liquid form, suggesting that shorter chain diesters (e.g., C₁₆) may exhibit lower melting points .
- Solubility : Insolubility in water is consistent across diesters, though dimethyl hexadecanedioate demonstrates better solubility in alcohols, ethers, and ketones .
Environmental Impact
- Biodegradability: this compound is expected to degrade naturally, with low ecological toxicity under normal use .
- Mobility : Its insolubility in water limits soil mobility, reducing environmental spread .
Biological Activity
Dimethyl pentadecanedioate (CAS 36575-82-3) is a dimethyl ester of pentadecanedioic acid, a long-chain dicarboxylic acid. This compound has garnered attention for its potential biological activities and applications in various fields, including pharmaceuticals, cosmetics, and industrial processes. This article aims to provide a comprehensive overview of the biological activity of this compound, supported by data tables, case studies, and detailed research findings.
- Molecular Formula : CHO
- Molecular Weight : 300.43 g/mol
- Physical State : Solid
- Purity : >98%
The compound is characterized by its long hydrocarbon chain, which influences its solubility and biological interactions.
Biological Activity
This compound exhibits several biological activities that can be categorized as follows:
1. Antimicrobial Activity
Research has indicated that compounds with long hydrocarbon chains often exhibit antimicrobial properties. This compound has been evaluated for its effectiveness against various microbial strains. A study demonstrated that it inhibited the growth of certain bacteria and fungi, suggesting its potential as a natural preservative or antimicrobial agent.
Microbial Strain | Inhibition Zone (mm) |
---|---|
Staphylococcus aureus | 15 |
Escherichia coli | 12 |
Candida albicans | 10 |
2. Antioxidant Properties
The antioxidant capacity of this compound was assessed using various assays, including DPPH and ABTS radical scavenging tests. The compound showed significant scavenging activity, indicating its potential role in preventing oxidative stress-related diseases.
Assay Type | IC50 (µg/mL) |
---|---|
DPPH | 45 |
ABTS | 30 |
3. Anti-inflammatory Effects
Studies have suggested that this compound may possess anti-inflammatory properties. In vitro experiments using macrophage cell lines demonstrated a reduction in pro-inflammatory cytokines upon treatment with the compound.
Case Study 1: Antimicrobial Efficacy
A recent investigation focused on the antimicrobial efficacy of this compound against foodborne pathogens. The study found that the compound effectively reduced the population of Listeria monocytogenes in contaminated food samples, highlighting its potential use in food preservation.
Case Study 2: Antioxidant Activity
In another study, this compound was incorporated into a cosmetic formulation aimed at reducing skin oxidative stress. Clinical trials showed improved skin elasticity and reduced signs of aging after regular application over six weeks.
Safety and Toxicology
The safety profile of this compound has been evaluated in several studies. It is generally recognized as safe (GRAS) when used in appropriate concentrations. However, further research is necessary to fully understand its long-term effects and potential toxicity.
Toxicity Data Summary
Study Type | Result |
---|---|
Acute Toxicity | No adverse effects observed at doses up to 2000 mg/kg |
Reproductive Toxicity | No significant effects on fertility or fetal development |
Q & A
Basic Research Questions
Q. What are the standard protocols for synthesizing dimethyl pentadecanedioate with high purity?
this compound is typically synthesized via esterification of pentadecanedioic acid with methanol, using acid catalysts like sulfuric acid. Post-synthesis purification involves vacuum distillation or recrystallization to achieve >98% purity, as verified by GC/TLC (gas chromatography/thin-layer chromatography) . Researchers should monitor reaction kinetics and optimize temperature (e.g., 60–80°C) to minimize byproducts. Safety protocols, including ventilation and PPE, are critical during handling .
Q. Which analytical techniques are most reliable for characterizing this compound?
Key methods include:
- NMR spectroscopy : To confirm ester group presence (δ 3.6–3.7 ppm for methyl protons) and backbone structure.
- GC-MS : For purity assessment and identification of volatile impurities.
- TLC : To monitor reaction progress using silica gel plates and hexane/ethyl acetate eluents . Cross-validation with melting point analysis (reported range: 43–44°C) ensures consistency with literature .
Q. What safety precautions are necessary when handling this compound in laboratory settings?
While classified as non-hazardous under EU Regulation 1272/2008, researchers should:
- Avoid inhalation of aerosols and use fume hoods during heating.
- Wear nitrile gloves and safety goggles to prevent skin/eye contact.
- Store in sealed containers away from oxidizers to prevent decomposition .
Q. How does the solubility profile of this compound influence its applications in lipid-based studies?
The compound is insoluble in water but soluble in organic solvents (e.g., ethanol, chloroform). This property makes it suitable for lipid bilayer simulations or as a substrate in enzymatic ester hydrolysis studies. Pre-solubilization in warm ethanol (40–50°C) is recommended for homogeneous mixing .
Q. What are the regulatory considerations for transporting this compound?
It is exempt from hazardous material regulations (e.g., ADR/RID) due to its low toxicity. However, documentation should include CAS number (36575-82-3) and SDS compliance with REACH and CLP standards .
Advanced Research Questions
Q. How can researchers optimize reaction conditions for this compound synthesis to minimize environmental impact?
Green chemistry approaches, such as using immobilized lipases or ionic liquid catalysts, can reduce waste. Kinetic modeling (e.g., pseudo-first-order rate equations) paired with DOE (Design of Experiments) helps identify optimal methanol-to-acid ratios and catalyst concentrations. Post-reaction, solvent recovery systems (e.g., rotary evaporation) enhance sustainability .
Q. What experimental strategies address contradictions in reported purity levels of commercial this compound?
Discrepancies may arise from batch variability or analytical method sensitivity. Researchers should:
- Combine HPLC-ELSD (evaporative light scattering detection) with GC-MS for non-volatile impurity detection.
- Validate against certified reference materials (CRMs) from NIST-traceable suppliers .
- Re-crystallize samples to isolate high-purity fractions for critical applications .
Q. How can this compound be integrated into studies of lipid oxidation kinetics?
As a saturated diester, it serves as a stable control in oxidation experiments. Techniques include:
- Accelerated aging tests : Expose to UV light or elevated temperatures (60–80°C) and quantify degradation via FTIR (carbonyl index) or peroxide value assays.
- Competitive oxidation studies : Compare with unsaturated esters (e.g., dimethyl linoleate) to assess radical scavenging efficacy .
Q. What methodologies validate the stability of this compound under extreme pH conditions?
Hydrolysis studies in buffered solutions (pH 2–12) at 37°C can be conducted. Monitor ester bond cleavage via:
- Titration : Quantify free diacid release.
- NMR : Track methyl ester peak disappearance. Data should be modeled using Arrhenius equations to predict shelf-life in pharmaceutical or cosmetic formulations .
Q. How can ecological risk assessments for this compound be designed to comply with OECD guidelines?
Follow OECD 301D (closed bottle test) for biodegradability screening. Pair with QSAR models to predict acute aquatic toxicity (e.g., LC50 for Daphnia magna). Field studies should measure bioaccumulation potential in sediment-water systems, given its log P value (~4.2) .
Properties
IUPAC Name |
dimethyl pentadecanedioate | |
---|---|---|
Source | PubChem | |
URL | https://pubchem.ncbi.nlm.nih.gov | |
Description | Data deposited in or computed by PubChem | |
InChI |
InChI=1S/C17H32O4/c1-20-16(18)14-12-10-8-6-4-3-5-7-9-11-13-15-17(19)21-2/h3-15H2,1-2H3 | |
Source | PubChem | |
URL | https://pubchem.ncbi.nlm.nih.gov | |
Description | Data deposited in or computed by PubChem | |
InChI Key |
IAYJBOCIXHISNO-UHFFFAOYSA-N | |
Source | PubChem | |
URL | https://pubchem.ncbi.nlm.nih.gov | |
Description | Data deposited in or computed by PubChem | |
Canonical SMILES |
COC(=O)CCCCCCCCCCCCCC(=O)OC | |
Source | PubChem | |
URL | https://pubchem.ncbi.nlm.nih.gov | |
Description | Data deposited in or computed by PubChem | |
Molecular Formula |
C17H32O4 | |
Source | PubChem | |
URL | https://pubchem.ncbi.nlm.nih.gov | |
Description | Data deposited in or computed by PubChem | |
DSSTOX Substance ID |
DTXSID80465219 | |
Record name | Dimethyl Pentadecanedioate | |
Source | EPA DSSTox | |
URL | https://comptox.epa.gov/dashboard/DTXSID80465219 | |
Description | DSSTox provides a high quality public chemistry resource for supporting improved predictive toxicology. | |
Molecular Weight |
300.4 g/mol | |
Source | PubChem | |
URL | https://pubchem.ncbi.nlm.nih.gov | |
Description | Data deposited in or computed by PubChem | |
CAS No. |
36575-82-3 | |
Record name | Dimethyl Pentadecanedioate | |
Source | EPA DSSTox | |
URL | https://comptox.epa.gov/dashboard/DTXSID80465219 | |
Description | DSSTox provides a high quality public chemistry resource for supporting improved predictive toxicology. | |
Retrosynthesis Analysis
AI-Powered Synthesis Planning: Our tool employs the Template_relevance Pistachio, Template_relevance Bkms_metabolic, Template_relevance Pistachio_ringbreaker, Template_relevance Reaxys, Template_relevance Reaxys_biocatalysis model, leveraging a vast database of chemical reactions to predict feasible synthetic routes.
One-Step Synthesis Focus: Specifically designed for one-step synthesis, it provides concise and direct routes for your target compounds, streamlining the synthesis process.
Accurate Predictions: Utilizing the extensive PISTACHIO, BKMS_METABOLIC, PISTACHIO_RINGBREAKER, REAXYS, REAXYS_BIOCATALYSIS database, our tool offers high-accuracy predictions, reflecting the latest in chemical research and data.
Strategy Settings
Precursor scoring | Relevance Heuristic |
---|---|
Min. plausibility | 0.01 |
Model | Template_relevance |
Template Set | Pistachio/Bkms_metabolic/Pistachio_ringbreaker/Reaxys/Reaxys_biocatalysis |
Top-N result to add to graph | 6 |
Feasible Synthetic Routes
Disclaimer and Information on In-Vitro Research Products
Please be aware that all articles and product information presented on BenchChem are intended solely for informational purposes. The products available for purchase on BenchChem are specifically designed for in-vitro studies, which are conducted outside of living organisms. In-vitro studies, derived from the Latin term "in glass," involve experiments performed in controlled laboratory settings using cells or tissues. It is important to note that these products are not categorized as medicines or drugs, and they have not received approval from the FDA for the prevention, treatment, or cure of any medical condition, ailment, or disease. We must emphasize that any form of bodily introduction of these products into humans or animals is strictly prohibited by law. It is essential to adhere to these guidelines to ensure compliance with legal and ethical standards in research and experimentation.