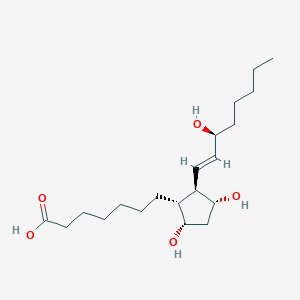
Prostaglandin f1alpha
Overview
Description
Prostaglandin F1alpha, also known as 6-keto-prostaglandin F1alpha, is a stable metabolite of prostacyclin (prostaglandin I2). It is a physiologically active compound found in very small amounts in all mammals. In human plasma, concentrations of this compound are typically less than 50 picograms per milliliter . This compound plays a significant role in various physiological processes, including vasodilation, inhibition of platelet aggregation, and modulation of inflammatory responses .
Mechanism of Action
Target of Action:
PGF1α primarily targets prostanoid receptors , which are G-protein-coupled receptors (GPCRs). These receptors are expressed in various tissues and play a crucial role in mediating the effects of prostaglandins. In particular, PGF1α interacts with the DP1 receptor (also known as the FP receptor ), which is found in smooth muscle cells, endothelial cells, and other tissues . The DP1 receptor is involved in regulating smooth muscle contraction and other physiological processes.
Mode of Action:
When PGF1α binds to the DP1 receptor, it activates downstream signaling pathways. Specifically:
- Smooth Muscle Contraction : PGF1α binding to the DP1 receptor promotes smooth muscle contraction, affecting blood vessels, bronchioles, and other smooth muscle-containing structures .
- Inflammation and Vascular Responses : PGF1α contributes to inflammation by influencing vascular matrix remodeling, endothelial cell apoptosis, and proliferation. It also plays a role in vasoconstriction and vascular smooth muscle cell proliferation and hypertrophy .
Action Environment:
Environmental factors, such as tissue oxygen levels, pH, and local concentrations of other signaling molecules, can influence PGF1α’s efficacy and stability. For instance, hypoxia may enhance its effects on vasodilation.
Biochemical Analysis
Biochemical Properties
Prostaglandin F1alpha is involved in a host of biological research areas due to its role in vascular and smooth muscle activity . It is often studied for its effects on vasodilation and the modulation of blood flow . It is also of interest in studies investigating smooth muscle contraction, where it provides insights into the mechanisms governing gastrointestinal, uterine, and bronchial function .
Cellular Effects
This compound controls a wide range of biological processes from blood pressure homeostasis to inflammation and resolution thereof to the perception of pain to cell survival . Disruption of normal prostanoid signaling, which includes this compound, is implicated in numerous disease states .
Molecular Mechanism
This compound signaling is facilitated by G-protein-coupled, prostanoid-specific receptors and the array of associated G-proteins . This review focuses on the expression, characterization, regulation, and mechanism of action of prostanoid receptors with particular emphasis on human isoforms .
Temporal Effects in Laboratory Settings
This compound is derived mainly from Prostaglandin E1, and is metabolized to 6-Keto this compound . This compound is excreted directly into the urine . This compound contracts the circular muscle of the gut in opposition to the Prostaglandins of the E series .
Dosage Effects in Animal Models
In veterinary science, prostaglandins including this compound have been used as agents capable of affecting the breeding and treatment of infertility in larger domestic species . The effects of this compound vary with different dosages in animal models .
Metabolic Pathways
This compound is a putative metabolite of dihomo-gamma-linolenic acid (DGLA) via the cyclooxygenase (COX) pathway . Both this compound and PGF2alpha have been shown to act as priming pheromones for male Atlantic salmon with a threshold concentration of 10-11 M .
Preparation Methods
Synthetic Routes and Reaction Conditions
Prostaglandin F1alpha can be synthesized through the non-enzymatic hydrolysis of prostacyclin (prostaglandin I2). The production of prostacyclin is typically monitored by measuring the levels of this compound, as prostacyclin has a very short half-life in plasma (approximately 60 minutes) and even shorter in buffer (2 to 3 minutes) . The synthetic route involves the hydration of prostacyclin under controlled conditions to yield this compound.
Industrial Production Methods
Industrial production of this compound involves the use of high-performance liquid chromatography (HPLC), gas chromatography/mass spectrometry (GC/MS), radioimmunoassay, or enzyme immunoassay techniques to measure and isolate the compound from biological fluids such as serum, urine, and tissue culture media .
Chemical Reactions Analysis
Types of Reactions
Prostaglandin F1alpha undergoes various chemical reactions, including:
Oxidation: This compound can be oxidized to form oxidized phosphatidic acids, such as PA(PGF1alpha/2:0).
Reduction: The compound can be reduced to form different metabolites, including tetranor-prostaglandin F1alpha.
Substitution: this compound can participate in substitution reactions, where functional groups are replaced by other groups.
Common Reagents and Conditions
Common reagents used in the reactions of this compound include oxidizing agents, reducing agents, and various catalysts. The conditions for these reactions typically involve controlled temperatures, pH levels, and the presence of specific solvents to facilitate the desired transformations.
Major Products Formed
The major products formed from the reactions of this compound include oxidized phosphatidic acids, reduced metabolites such as tetranor-prostaglandin F1alpha, and various substituted derivatives .
Scientific Research Applications
Prostaglandin F1alpha has a wide range of scientific research applications, including:
Comparison with Similar Compounds
Similar Compounds
Prostaglandin F2alpha: Another member of the prostaglandin family, known for its role in smooth muscle contraction and regulation of reproductive functions.
Prostaglandin D2: Involved in the regulation of sleep, allergic responses, and inflammation.
Prostaglandin E1: Known for its vasodilatory and anti-inflammatory properties.
Uniqueness of Prostaglandin F1alpha
This compound is unique due to its stable nature as a metabolite of prostacyclin and its significant role in vasodilation and inhibition of platelet aggregation . Unlike other prostaglandins, this compound is specifically used as a marker for prostacyclin production and has distinct clinical applications in cardiovascular health .
Properties
IUPAC Name |
7-[(1R,2R,3R,5S)-3,5-dihydroxy-2-[(E,3S)-3-hydroxyoct-1-enyl]cyclopentyl]heptanoic acid | |
---|---|---|
Source | PubChem | |
URL | https://pubchem.ncbi.nlm.nih.gov | |
Description | Data deposited in or computed by PubChem | |
InChI |
InChI=1S/C20H36O5/c1-2-3-6-9-15(21)12-13-17-16(18(22)14-19(17)23)10-7-4-5-8-11-20(24)25/h12-13,15-19,21-23H,2-11,14H2,1H3,(H,24,25)/b13-12+/t15-,16+,17+,18-,19+/m0/s1 | |
Source | PubChem | |
URL | https://pubchem.ncbi.nlm.nih.gov | |
Description | Data deposited in or computed by PubChem | |
InChI Key |
DZUXGQBLFALXCR-CDIPTNKSSA-N | |
Source | PubChem | |
URL | https://pubchem.ncbi.nlm.nih.gov | |
Description | Data deposited in or computed by PubChem | |
Canonical SMILES |
CCCCCC(C=CC1C(CC(C1CCCCCCC(=O)O)O)O)O | |
Source | PubChem | |
URL | https://pubchem.ncbi.nlm.nih.gov | |
Description | Data deposited in or computed by PubChem | |
Isomeric SMILES |
CCCCC[C@@H](/C=C/[C@H]1[C@@H](C[C@@H]([C@@H]1CCCCCCC(=O)O)O)O)O | |
Source | PubChem | |
URL | https://pubchem.ncbi.nlm.nih.gov | |
Description | Data deposited in or computed by PubChem | |
Molecular Formula |
C20H36O5 | |
Source | PubChem | |
URL | https://pubchem.ncbi.nlm.nih.gov | |
Description | Data deposited in or computed by PubChem | |
DSSTOX Substance ID |
DTXSID901339475 | |
Record name | (9alpha,11alpha,13E,15S)-9,11,15-Trihydroxyprost-13-en-1-oic acid | |
Source | EPA DSSTox | |
URL | https://comptox.epa.gov/dashboard/DTXSID901339475 | |
Description | DSSTox provides a high quality public chemistry resource for supporting improved predictive toxicology. | |
Molecular Weight |
356.5 g/mol | |
Source | PubChem | |
URL | https://pubchem.ncbi.nlm.nih.gov | |
Description | Data deposited in or computed by PubChem | |
Physical Description |
Solid | |
Record name | Prostaglandin F1a | |
Source | Human Metabolome Database (HMDB) | |
URL | http://www.hmdb.ca/metabolites/HMDB0002685 | |
Description | The Human Metabolome Database (HMDB) is a freely available electronic database containing detailed information about small molecule metabolites found in the human body. | |
Explanation | HMDB is offered to the public as a freely available resource. Use and re-distribution of the data, in whole or in part, for commercial purposes requires explicit permission of the authors and explicit acknowledgment of the source material (HMDB) and the original publication (see the HMDB citing page). We ask that users who download significant portions of the database cite the HMDB paper in any resulting publications. | |
CAS No. |
745-62-0 | |
Record name | PGF1α | |
Source | CAS Common Chemistry | |
URL | https://commonchemistry.cas.org/detail?cas_rn=745-62-0 | |
Description | CAS Common Chemistry is an open community resource for accessing chemical information. Nearly 500,000 chemical substances from CAS REGISTRY cover areas of community interest, including common and frequently regulated chemicals, and those relevant to high school and undergraduate chemistry classes. This chemical information, curated by our expert scientists, is provided in alignment with our mission as a division of the American Chemical Society. | |
Explanation | The data from CAS Common Chemistry is provided under a CC-BY-NC 4.0 license, unless otherwise stated. | |
Record name | Prostaglandin F1 | |
Source | ChemIDplus | |
URL | https://pubchem.ncbi.nlm.nih.gov/substance/?source=chemidplus&sourceid=0000745620 | |
Description | ChemIDplus is a free, web search system that provides access to the structure and nomenclature authority files used for the identification of chemical substances cited in National Library of Medicine (NLM) databases, including the TOXNET system. | |
Record name | (9alpha,11alpha,13E,15S)-9,11,15-Trihydroxyprost-13-en-1-oic acid | |
Source | EPA DSSTox | |
URL | https://comptox.epa.gov/dashboard/DTXSID901339475 | |
Description | DSSTox provides a high quality public chemistry resource for supporting improved predictive toxicology. | |
Record name | PROSTAGLANDIN F1 | |
Source | FDA Global Substance Registration System (GSRS) | |
URL | https://gsrs.ncats.nih.gov/ginas/app/beta/substances/WJ72O6860W | |
Description | The FDA Global Substance Registration System (GSRS) enables the efficient and accurate exchange of information on what substances are in regulated products. Instead of relying on names, which vary across regulatory domains, countries, and regions, the GSRS knowledge base makes it possible for substances to be defined by standardized, scientific descriptions. | |
Explanation | Unless otherwise noted, the contents of the FDA website (www.fda.gov), both text and graphics, are not copyrighted. They are in the public domain and may be republished, reprinted and otherwise used freely by anyone without the need to obtain permission from FDA. Credit to the U.S. Food and Drug Administration as the source is appreciated but not required. | |
Record name | Prostaglandin F1a | |
Source | Human Metabolome Database (HMDB) | |
URL | http://www.hmdb.ca/metabolites/HMDB0002685 | |
Description | The Human Metabolome Database (HMDB) is a freely available electronic database containing detailed information about small molecule metabolites found in the human body. | |
Explanation | HMDB is offered to the public as a freely available resource. Use and re-distribution of the data, in whole or in part, for commercial purposes requires explicit permission of the authors and explicit acknowledgment of the source material (HMDB) and the original publication (see the HMDB citing page). We ask that users who download significant portions of the database cite the HMDB paper in any resulting publications. | |
Retrosynthesis Analysis
AI-Powered Synthesis Planning: Our tool employs the Template_relevance Pistachio, Template_relevance Bkms_metabolic, Template_relevance Pistachio_ringbreaker, Template_relevance Reaxys, Template_relevance Reaxys_biocatalysis model, leveraging a vast database of chemical reactions to predict feasible synthetic routes.
One-Step Synthesis Focus: Specifically designed for one-step synthesis, it provides concise and direct routes for your target compounds, streamlining the synthesis process.
Accurate Predictions: Utilizing the extensive PISTACHIO, BKMS_METABOLIC, PISTACHIO_RINGBREAKER, REAXYS, REAXYS_BIOCATALYSIS database, our tool offers high-accuracy predictions, reflecting the latest in chemical research and data.
Strategy Settings
Precursor scoring | Relevance Heuristic |
---|---|
Min. plausibility | 0.01 |
Model | Template_relevance |
Template Set | Pistachio/Bkms_metabolic/Pistachio_ringbreaker/Reaxys/Reaxys_biocatalysis |
Top-N result to add to graph | 6 |
Feasible Synthetic Routes
Disclaimer and Information on In-Vitro Research Products
Please be aware that all articles and product information presented on BenchChem are intended solely for informational purposes. The products available for purchase on BenchChem are specifically designed for in-vitro studies, which are conducted outside of living organisms. In-vitro studies, derived from the Latin term "in glass," involve experiments performed in controlled laboratory settings using cells or tissues. It is important to note that these products are not categorized as medicines or drugs, and they have not received approval from the FDA for the prevention, treatment, or cure of any medical condition, ailment, or disease. We must emphasize that any form of bodily introduction of these products into humans or animals is strictly prohibited by law. It is essential to adhere to these guidelines to ensure compliance with legal and ethical standards in research and experimentation.