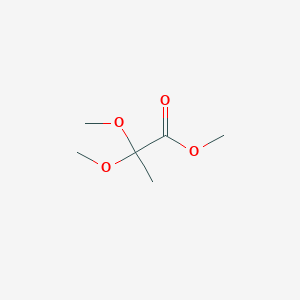
Methyl 2,2-dimethoxypropanoate
Overview
Description
Methyl 2,2-dimethoxypropanoate is an organic compound with the molecular formula C6H12O4. It is a colorless liquid that is used in various chemical reactions and industrial applications. The compound is known for its stability and reactivity, making it a valuable intermediate in organic synthesis.
Preparation Methods
Synthetic Routes and Reaction Conditions: Methyl 2,2-dimethoxypropanoate can be synthesized through the reaction of methyl pyruvate with trimethyl orthoformate in the presence of a catalytic amount of concentrated sulfuric acid. The reaction is typically carried out in methanol under reflux conditions for several hours. The reaction mixture is then cooled, and the product is isolated by distillation .
Industrial Production Methods: In industrial settings, the preparation of this compound involves similar reaction conditions but on a larger scale. The use of continuous flow reactors and optimized reaction parameters can enhance the yield and purity of the product. The crude product is often purified through distillation or recrystallization to achieve the desired quality.
Chemical Reactions Analysis
Types of Reactions: Methyl 2,2-dimethoxypropanoate undergoes various chemical reactions, including:
Hydrolysis: The ester group can be hydrolyzed to produce the corresponding carboxylic acid and methanol.
Reduction: The compound can be reduced to yield the corresponding alcohol.
Substitution: The methoxy groups can be substituted with other nucleophiles under appropriate conditions.
Common Reagents and Conditions:
Hydrolysis: Acidic or basic conditions, typically using hydrochloric acid or sodium hydroxide.
Reduction: Lithium aluminum hydride (LiAlH4) or sodium borohydride (NaBH4) in anhydrous solvents.
Substitution: Nucleophiles such as amines or thiols in the presence of a suitable catalyst.
Major Products Formed:
Hydrolysis: Propanoic acid and methanol.
Reduction: 2,2-dimethoxypropanol.
Substitution: Various substituted derivatives depending on the nucleophile used.
Scientific Research Applications
Methyl 2,2-dimethoxypropanoate is utilized in several scientific research applications, including:
Organic Synthesis: As an intermediate in the synthesis of complex organic molecules.
Pharmaceuticals: In the development of drug candidates and active pharmaceutical ingredients.
Materials Science: As a precursor for the synthesis of polymers and advanced materials.
Biological Studies: In the study of enzyme-catalyzed reactions and metabolic pathways.
Mechanism of Action
The mechanism of action of methyl 2,2-dimethoxypropanoate involves its reactivity as an ester and its ability to undergo various chemical transformations. The compound can act as a substrate for esterases and other enzymes, leading to the formation of reactive intermediates. These intermediates can participate in further chemical reactions, contributing to the compound’s versatility in organic synthesis.
Comparison with Similar Compounds
- Methyl 2,2-dimethoxypropionate
- Ethyl 2,2-dimethoxypropanoate
- Methyl 2,2-dimethoxybutanoate
Comparison: Methyl 2,2-dimethoxypropanoate is unique due to its specific molecular structure, which imparts distinct reactivity and stability. Compared to similar compounds, it offers a balance of reactivity and ease of handling, making it a preferred choice in various synthetic applications. Its methoxy groups provide steric hindrance, influencing the compound’s reactivity and selectivity in chemical reactions.
Properties
IUPAC Name |
methyl 2,2-dimethoxypropanoate | |
---|---|---|
Source | PubChem | |
URL | https://pubchem.ncbi.nlm.nih.gov | |
Description | Data deposited in or computed by PubChem | |
InChI |
InChI=1S/C6H12O4/c1-6(9-3,10-4)5(7)8-2/h1-4H3 | |
Source | PubChem | |
URL | https://pubchem.ncbi.nlm.nih.gov | |
Description | Data deposited in or computed by PubChem | |
InChI Key |
KHQQDBIXHUJARJ-UHFFFAOYSA-N | |
Source | PubChem | |
URL | https://pubchem.ncbi.nlm.nih.gov | |
Description | Data deposited in or computed by PubChem | |
Canonical SMILES |
CC(C(=O)OC)(OC)OC | |
Source | PubChem | |
URL | https://pubchem.ncbi.nlm.nih.gov | |
Description | Data deposited in or computed by PubChem | |
Molecular Formula |
C6H12O4 | |
Source | PubChem | |
URL | https://pubchem.ncbi.nlm.nih.gov | |
Description | Data deposited in or computed by PubChem | |
DSSTOX Substance ID |
DTXSID60143503 | |
Record name | Methyl 2,2-dimethoxypropionate | |
Source | EPA DSSTox | |
URL | https://comptox.epa.gov/dashboard/DTXSID60143503 | |
Description | DSSTox provides a high quality public chemistry resource for supporting improved predictive toxicology. | |
Molecular Weight |
148.16 g/mol | |
Source | PubChem | |
URL | https://pubchem.ncbi.nlm.nih.gov | |
Description | Data deposited in or computed by PubChem | |
CAS No. |
10076-48-9 | |
Record name | Methyl 2,2-dimethoxypropionate | |
Source | CAS Common Chemistry | |
URL | https://commonchemistry.cas.org/detail?cas_rn=10076-48-9 | |
Description | CAS Common Chemistry is an open community resource for accessing chemical information. Nearly 500,000 chemical substances from CAS REGISTRY cover areas of community interest, including common and frequently regulated chemicals, and those relevant to high school and undergraduate chemistry classes. This chemical information, curated by our expert scientists, is provided in alignment with our mission as a division of the American Chemical Society. | |
Explanation | The data from CAS Common Chemistry is provided under a CC-BY-NC 4.0 license, unless otherwise stated. | |
Record name | Methyl 2,2-dimethoxypropionate | |
Source | ChemIDplus | |
URL | https://pubchem.ncbi.nlm.nih.gov/substance/?source=chemidplus&sourceid=0010076489 | |
Description | ChemIDplus is a free, web search system that provides access to the structure and nomenclature authority files used for the identification of chemical substances cited in National Library of Medicine (NLM) databases, including the TOXNET system. | |
Record name | 10076-48-9 | |
Source | DTP/NCI | |
URL | https://dtp.cancer.gov/dtpstandard/servlet/dwindex?searchtype=NSC&outputformat=html&searchlist=159637 | |
Description | The NCI Development Therapeutics Program (DTP) provides services and resources to the academic and private-sector research communities worldwide to facilitate the discovery and development of new cancer therapeutic agents. | |
Explanation | Unless otherwise indicated, all text within NCI products is free of copyright and may be reused without our permission. Credit the National Cancer Institute as the source. | |
Record name | Methyl 2,2-dimethoxypropionate | |
Source | EPA DSSTox | |
URL | https://comptox.epa.gov/dashboard/DTXSID60143503 | |
Description | DSSTox provides a high quality public chemistry resource for supporting improved predictive toxicology. | |
Record name | Methyl 2,2-dimethoxypropionate | |
Source | European Chemicals Agency (ECHA) | |
URL | https://echa.europa.eu/substance-information/-/substanceinfo/100.030.179 | |
Description | The European Chemicals Agency (ECHA) is an agency of the European Union which is the driving force among regulatory authorities in implementing the EU's groundbreaking chemicals legislation for the benefit of human health and the environment as well as for innovation and competitiveness. | |
Explanation | Use of the information, documents and data from the ECHA website is subject to the terms and conditions of this Legal Notice, and subject to other binding limitations provided for under applicable law, the information, documents and data made available on the ECHA website may be reproduced, distributed and/or used, totally or in part, for non-commercial purposes provided that ECHA is acknowledged as the source: "Source: European Chemicals Agency, http://echa.europa.eu/". Such acknowledgement must be included in each copy of the material. ECHA permits and encourages organisations and individuals to create links to the ECHA website under the following cumulative conditions: Links can only be made to webpages that provide a link to the Legal Notice page. | |
Retrosynthesis Analysis
AI-Powered Synthesis Planning: Our tool employs the Template_relevance Pistachio, Template_relevance Bkms_metabolic, Template_relevance Pistachio_ringbreaker, Template_relevance Reaxys, Template_relevance Reaxys_biocatalysis model, leveraging a vast database of chemical reactions to predict feasible synthetic routes.
One-Step Synthesis Focus: Specifically designed for one-step synthesis, it provides concise and direct routes for your target compounds, streamlining the synthesis process.
Accurate Predictions: Utilizing the extensive PISTACHIO, BKMS_METABOLIC, PISTACHIO_RINGBREAKER, REAXYS, REAXYS_BIOCATALYSIS database, our tool offers high-accuracy predictions, reflecting the latest in chemical research and data.
Strategy Settings
Precursor scoring | Relevance Heuristic |
---|---|
Min. plausibility | 0.01 |
Model | Template_relevance |
Template Set | Pistachio/Bkms_metabolic/Pistachio_ringbreaker/Reaxys/Reaxys_biocatalysis |
Top-N result to add to graph | 6 |
Feasible Synthetic Routes
Disclaimer and Information on In-Vitro Research Products
Please be aware that all articles and product information presented on BenchChem are intended solely for informational purposes. The products available for purchase on BenchChem are specifically designed for in-vitro studies, which are conducted outside of living organisms. In-vitro studies, derived from the Latin term "in glass," involve experiments performed in controlled laboratory settings using cells or tissues. It is important to note that these products are not categorized as medicines or drugs, and they have not received approval from the FDA for the prevention, treatment, or cure of any medical condition, ailment, or disease. We must emphasize that any form of bodily introduction of these products into humans or animals is strictly prohibited by law. It is essential to adhere to these guidelines to ensure compliance with legal and ethical standards in research and experimentation.