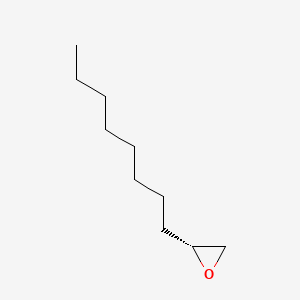
(R)-1,2-Epoxydecane
Overview
Description
®-1,2-Epoxydecane is an organic compound belonging to the class of epoxides. It is a chiral molecule, meaning it has a non-superimposable mirror image. The compound is characterized by a three-membered cyclic ether structure, where an oxygen atom is bonded to two adjacent carbon atoms. This structure imparts significant reactivity to the molecule, making it a valuable intermediate in various chemical processes.
Preparation Methods
Synthetic Routes and Reaction Conditions: ®-1,2-Epoxydecane can be synthesized through several methods, including:
Epoxidation of Alkenes: One common method involves the epoxidation of 1-decene using peracids such as meta-chloroperoxybenzoic acid (mCPBA) under mild conditions. The reaction proceeds via the formation of an oxirane ring.
Halohydrin Formation and Cyclization: Another method involves the formation of a halohydrin intermediate from 1-decene, followed by intramolecular cyclization to form the epoxide. This can be achieved using reagents like hypochlorous acid (HOCl) or hypobromous acid (HOBr).
Industrial Production Methods: In industrial settings, the production of ®-1,2-Epoxydecane often involves the use of large-scale epoxidation processes. These processes typically employ catalysts such as titanium silicalite-1 (TS-1) in the presence of hydrogen peroxide (H2O2) to achieve high yields and selectivity.
Chemical Reactions Analysis
Types of Reactions: ®-1,2-Epoxydecane undergoes various chemical reactions, including:
Nucleophilic Substitution: The strained three-membered ring of the epoxide is highly susceptible to nucleophilic attack, leading to ring-opening reactions. Common nucleophiles include water, alcohols, and amines.
Reduction: The compound can be reduced to the corresponding diol using reducing agents such as lithium aluminum hydride (LiAlH4).
Oxidation: Although less common, ®-1,2-Epoxydecane can undergo oxidation to form more complex oxygenated products.
Common Reagents and Conditions:
Nucleophilic Substitution: Reagents like sodium hydroxide (NaOH) or potassium hydroxide (KOH) in aqueous or alcoholic solutions.
Reduction: Lithium aluminum hydride (LiAlH4) in anhydrous ether.
Oxidation: Strong oxidizing agents like potassium permanganate (KMnO4) or osmium tetroxide (OsO4).
Major Products:
Nucleophilic Substitution: The major products are typically 1,2-diols or other ring-opened derivatives.
Reduction: The major product is 1,2-decanediol.
Oxidation: The products vary depending on the oxidizing agent used but can include carboxylic acids or ketones.
Scientific Research Applications
®-1,2-Epoxydecane has a wide range of applications in scientific research, including:
Chemistry: It is used as an intermediate in the synthesis of various complex organic molecules, including pharmaceuticals and agrochemicals.
Biology: The compound is employed in studies involving enzyme-catalyzed epoxide ring-opening reactions, which are important in understanding metabolic pathways.
Medicine: ®-1,2-Epoxydecane derivatives are investigated for their potential therapeutic properties, including anti-inflammatory and anticancer activities.
Industry: It is used in the production of epoxy resins, which are essential components in coatings, adhesives, and composite materials.
Mechanism of Action
The mechanism of action of ®-1,2-Epoxydecane primarily involves its reactivity as an epoxide. The strained three-membered ring is prone to nucleophilic attack, leading to ring-opening reactions. These reactions can occur under both acidic and basic conditions, with the nucleophile attacking the less hindered carbon atom of the epoxide ring. The resulting products depend on the nature of the nucleophile and the reaction conditions.
Comparison with Similar Compounds
(S)-1,2-Epoxydecane: The enantiomer of ®-1,2-Epoxydecane, which has similar chemical properties but different biological activities due to its chiral nature.
1,2-Epoxyhexane: A shorter-chain analog with similar reactivity but different physical properties.
1,2-Epoxyoctane: Another analog with a slightly shorter carbon chain, used in similar applications.
Uniqueness: ®-1,2-Epoxydecane is unique due to its specific chiral configuration, which can impart distinct biological activities compared to its enantiomer and other epoxides. Its longer carbon chain also provides different solubility and reactivity characteristics compared to shorter-chain epoxides.
Properties
IUPAC Name |
(2R)-2-octyloxirane | |
---|---|---|
Source | PubChem | |
URL | https://pubchem.ncbi.nlm.nih.gov | |
Description | Data deposited in or computed by PubChem | |
InChI |
InChI=1S/C10H20O/c1-2-3-4-5-6-7-8-10-9-11-10/h10H,2-9H2,1H3/t10-/m1/s1 | |
Source | PubChem | |
URL | https://pubchem.ncbi.nlm.nih.gov | |
Description | Data deposited in or computed by PubChem | |
InChI Key |
AAMHBRRZYSORSH-SNVBAGLBSA-N | |
Source | PubChem | |
URL | https://pubchem.ncbi.nlm.nih.gov | |
Description | Data deposited in or computed by PubChem | |
Canonical SMILES |
CCCCCCCCC1CO1 | |
Source | PubChem | |
URL | https://pubchem.ncbi.nlm.nih.gov | |
Description | Data deposited in or computed by PubChem | |
Isomeric SMILES |
CCCCCCCC[C@@H]1CO1 | |
Source | PubChem | |
URL | https://pubchem.ncbi.nlm.nih.gov | |
Description | Data deposited in or computed by PubChem | |
Molecular Formula |
C10H20O | |
Source | PubChem | |
URL | https://pubchem.ncbi.nlm.nih.gov | |
Description | Data deposited in or computed by PubChem | |
Molecular Weight |
156.26 g/mol | |
Source | PubChem | |
URL | https://pubchem.ncbi.nlm.nih.gov | |
Description | Data deposited in or computed by PubChem | |
CAS No. |
67210-36-0 | |
Record name | 1,2-Epoxydecane, (+)- | |
Source | ChemIDplus | |
URL | https://pubchem.ncbi.nlm.nih.gov/substance/?source=chemidplus&sourceid=0067210360 | |
Description | ChemIDplus is a free, web search system that provides access to the structure and nomenclature authority files used for the identification of chemical substances cited in National Library of Medicine (NLM) databases, including the TOXNET system. | |
Record name | 67210-36-0 | |
Source | European Chemicals Agency (ECHA) | |
URL | https://echa.europa.eu/information-on-chemicals | |
Description | The European Chemicals Agency (ECHA) is an agency of the European Union which is the driving force among regulatory authorities in implementing the EU's groundbreaking chemicals legislation for the benefit of human health and the environment as well as for innovation and competitiveness. | |
Explanation | Use of the information, documents and data from the ECHA website is subject to the terms and conditions of this Legal Notice, and subject to other binding limitations provided for under applicable law, the information, documents and data made available on the ECHA website may be reproduced, distributed and/or used, totally or in part, for non-commercial purposes provided that ECHA is acknowledged as the source: "Source: European Chemicals Agency, http://echa.europa.eu/". Such acknowledgement must be included in each copy of the material. ECHA permits and encourages organisations and individuals to create links to the ECHA website under the following cumulative conditions: Links can only be made to webpages that provide a link to the Legal Notice page. | |
Record name | 1,2-EPOXYDECANE, (+)- | |
Source | FDA Global Substance Registration System (GSRS) | |
URL | https://gsrs.ncats.nih.gov/ginas/app/beta/substances/0P528KWQ7H | |
Description | The FDA Global Substance Registration System (GSRS) enables the efficient and accurate exchange of information on what substances are in regulated products. Instead of relying on names, which vary across regulatory domains, countries, and regions, the GSRS knowledge base makes it possible for substances to be defined by standardized, scientific descriptions. | |
Explanation | Unless otherwise noted, the contents of the FDA website (www.fda.gov), both text and graphics, are not copyrighted. They are in the public domain and may be republished, reprinted and otherwise used freely by anyone without the need to obtain permission from FDA. Credit to the U.S. Food and Drug Administration as the source is appreciated but not required. | |
Synthesis routes and methods
Procedure details
Retrosynthesis Analysis
AI-Powered Synthesis Planning: Our tool employs the Template_relevance Pistachio, Template_relevance Bkms_metabolic, Template_relevance Pistachio_ringbreaker, Template_relevance Reaxys, Template_relevance Reaxys_biocatalysis model, leveraging a vast database of chemical reactions to predict feasible synthetic routes.
One-Step Synthesis Focus: Specifically designed for one-step synthesis, it provides concise and direct routes for your target compounds, streamlining the synthesis process.
Accurate Predictions: Utilizing the extensive PISTACHIO, BKMS_METABOLIC, PISTACHIO_RINGBREAKER, REAXYS, REAXYS_BIOCATALYSIS database, our tool offers high-accuracy predictions, reflecting the latest in chemical research and data.
Strategy Settings
Precursor scoring | Relevance Heuristic |
---|---|
Min. plausibility | 0.01 |
Model | Template_relevance |
Template Set | Pistachio/Bkms_metabolic/Pistachio_ringbreaker/Reaxys/Reaxys_biocatalysis |
Top-N result to add to graph | 6 |
Feasible Synthetic Routes
Disclaimer and Information on In-Vitro Research Products
Please be aware that all articles and product information presented on BenchChem are intended solely for informational purposes. The products available for purchase on BenchChem are specifically designed for in-vitro studies, which are conducted outside of living organisms. In-vitro studies, derived from the Latin term "in glass," involve experiments performed in controlled laboratory settings using cells or tissues. It is important to note that these products are not categorized as medicines or drugs, and they have not received approval from the FDA for the prevention, treatment, or cure of any medical condition, ailment, or disease. We must emphasize that any form of bodily introduction of these products into humans or animals is strictly prohibited by law. It is essential to adhere to these guidelines to ensure compliance with legal and ethical standards in research and experimentation.