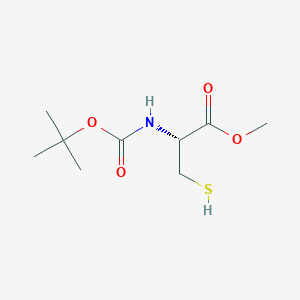
N-(tert-Butoxycarbonyl)-L-cysteine methyl ester
Overview
Description
N-(tert-Butoxycarbonyl)-L-cysteine methyl ester is an N-terminal protected reagent that can be used in the synthesis of peptides and proteins containing cysteine residues . It has a linear formula of HSCH2CH[NHCO2C(CH3)3]CO2CH3 and a molecular weight of 235.30 .
Synthesis Analysis
The tert-butyloxycarbonyl (Boc) group is one of the classical masking functionalities employed in organic synthesis for the protection of amino groups . The Boc-masking group is installed by reacting the amino-substrate with di-tert-butyl dicarbonate under basic conditions . Traditional approaches for N-Boc deprotection rely largely on TFA-induced cleavage .Molecular Structure Analysis
The molecular structure of N-(tert-Butoxycarbonyl)-L-cysteine methyl ester is represented by the SMILES string COC(=O)C@HNC(=O)OC©©C .Chemical Reactions Analysis
The reactions involving N-(tert-Butoxycarbonyl)-L-cysteine methyl ester take place under room temperature conditions for 1–4 h with yields up to 90% . This mild procedure was applied to a hybrid, medicinally active compound FC1, which is a novel dual inhibitor of IDO1 and DNA Pol gamma .Physical And Chemical Properties Analysis
N-(tert-Butoxycarbonyl)-L-cysteine methyl ester is a liquid at room temperature . It has an optical activity of [α]22/D +21°, c = 7.5 in chloroform . The refractive index is n20/D 1.475 (lit.) , and it has a boiling point of 214 °C (lit.) . The density is 1.143 g/mL at 25 °C (lit.) .Scientific Research Applications
Peptide Synthesis
“N-(tert-Butoxycarbonyl)-L-cysteine methyl ester” is commonly used as an N-terminal protected reagent in the synthesis of peptides and proteins containing cysteine residues. This protection is crucial for preventing unwanted side reactions during peptide coupling processes .
Protein Engineering
The compound can be utilized to introduce cysteine residues into peptides and proteins, which can then be converted to dehydroalanine. This conversion is significant for protein engineering applications, allowing for site-specific modifications .
Mechanism of Action
properties
IUPAC Name |
methyl (2R)-2-[(2-methylpropan-2-yl)oxycarbonylamino]-3-sulfanylpropanoate | |
---|---|---|
Source | PubChem | |
URL | https://pubchem.ncbi.nlm.nih.gov | |
Description | Data deposited in or computed by PubChem | |
InChI |
InChI=1S/C9H17NO4S/c1-9(2,3)14-8(12)10-6(5-15)7(11)13-4/h6,15H,5H2,1-4H3,(H,10,12)/t6-/m0/s1 | |
Source | PubChem | |
URL | https://pubchem.ncbi.nlm.nih.gov | |
Description | Data deposited in or computed by PubChem | |
InChI Key |
NJGIAKIPSDCYAC-LURJTMIESA-N | |
Source | PubChem | |
URL | https://pubchem.ncbi.nlm.nih.gov | |
Description | Data deposited in or computed by PubChem | |
Canonical SMILES |
CC(C)(C)OC(=O)NC(CS)C(=O)OC | |
Source | PubChem | |
URL | https://pubchem.ncbi.nlm.nih.gov | |
Description | Data deposited in or computed by PubChem | |
Isomeric SMILES |
CC(C)(C)OC(=O)N[C@@H](CS)C(=O)OC | |
Source | PubChem | |
URL | https://pubchem.ncbi.nlm.nih.gov | |
Description | Data deposited in or computed by PubChem | |
Molecular Formula |
C9H17NO4S | |
Source | PubChem | |
URL | https://pubchem.ncbi.nlm.nih.gov | |
Description | Data deposited in or computed by PubChem | |
DSSTOX Substance ID |
DTXSID60450522 | |
Record name | N-(tert-Butoxycarbonyl)-L-cysteine methyl ester | |
Source | EPA DSSTox | |
URL | https://comptox.epa.gov/dashboard/DTXSID60450522 | |
Description | DSSTox provides a high quality public chemistry resource for supporting improved predictive toxicology. | |
Molecular Weight |
235.30 g/mol | |
Source | PubChem | |
URL | https://pubchem.ncbi.nlm.nih.gov | |
Description | Data deposited in or computed by PubChem | |
Product Name |
N-(tert-Butoxycarbonyl)-L-cysteine methyl ester | |
CAS RN |
55757-46-5 | |
Record name | N-(tert-Butoxycarbonyl)-L-cysteine methyl ester | |
Source | EPA DSSTox | |
URL | https://comptox.epa.gov/dashboard/DTXSID60450522 | |
Description | DSSTox provides a high quality public chemistry resource for supporting improved predictive toxicology. | |
Retrosynthesis Analysis
AI-Powered Synthesis Planning: Our tool employs the Template_relevance Pistachio, Template_relevance Bkms_metabolic, Template_relevance Pistachio_ringbreaker, Template_relevance Reaxys, Template_relevance Reaxys_biocatalysis model, leveraging a vast database of chemical reactions to predict feasible synthetic routes.
One-Step Synthesis Focus: Specifically designed for one-step synthesis, it provides concise and direct routes for your target compounds, streamlining the synthesis process.
Accurate Predictions: Utilizing the extensive PISTACHIO, BKMS_METABOLIC, PISTACHIO_RINGBREAKER, REAXYS, REAXYS_BIOCATALYSIS database, our tool offers high-accuracy predictions, reflecting the latest in chemical research and data.
Strategy Settings
Precursor scoring | Relevance Heuristic |
---|---|
Min. plausibility | 0.01 |
Model | Template_relevance |
Template Set | Pistachio/Bkms_metabolic/Pistachio_ringbreaker/Reaxys/Reaxys_biocatalysis |
Top-N result to add to graph | 6 |
Feasible Synthetic Routes
Q & A
Q1: What makes N-(tert-Butoxycarbonyl)-L-cysteine methyl ester useful in materials science?
A1: This compound can be used as a building block for creating micropatterns of metallic nanoparticles. Specifically, it enables a process called photothermal-reaction-assisted two-photon direct laser writing. [] In this process, the compound acts as a thermally cleavable ligand for silver nanoparticles. When exposed to a pulsed laser, the heat triggers the cleavage of the ligand from the nanoparticle surface. This cleavage significantly changes the nanoparticles' dispersibility, allowing for a solvent-selective development process and the creation of precise, submicron-sized patterns. []
Q2: Can N-(tert-Butoxycarbonyl)-L-cysteine methyl ester be used to study chiral metabolites?
A2: Yes, N-(tert-Butoxycarbonyl)-L-cysteine methyl ester has shown promise as a chiral derivatizing agent for analyzing enantiomeric amine metabolites using liquid chromatography-mass spectrometry (LC-MS). [] While LC-MS is a powerful tool in metabolomics, differentiating between enantiomers remains challenging. This compound, when used in conjunction with o-phthalaldehyde (OPA), can form diastereomeric derivatives with chiral amines. These derivatives can then be separated and detected by LC-MS, allowing for the identification and quantification of individual enantiomers. []
Q3: How does N-(tert-Butoxycarbonyl)-L-cysteine methyl ester contribute to the synthesis of alliin analogues?
A3: This compound serves as a valuable precursor in the multi-step synthesis of alliin analogues, which are compounds with potential biological activity. [] Specifically, it can be chemically transformed into transient sulfenic acids. [] These sulfenic acids can react with various acceptors, ultimately yielding sulfoxides containing the desired alliin-like structure. This approach allows for the creation of enantiomerically pure alliin analogues, expanding the possibilities for investigating their biological properties. []
Q4: Can N-(tert-Butoxycarbonyl)-L-cysteine methyl ester be used in polymer chemistry?
A4: Yes, N-(tert-Butoxycarbonyl)-L-cysteine methyl ester can initiate the controlled ring-opening polymerization of its corresponding β-thiolactone. [] This polymerization leads to the formation of unique polythioesters. The thiol group of the compound acts as the initiator and the propagating species, attacking the carbonyl group of the β-thiolactone to open the ring. [] This process allows for the synthesis of well-defined polythioesters with specific chain lengths and functionalities. []
Q5: Are there analytical methods to detect N-(tert-Butoxycarbonyl)-L-cysteine methyl ester in biological samples?
A5: Although not directly mentioned in the context of N-(tert-Butoxycarbonyl)-L-cysteine methyl ester, a related compound, allyl isothiocyanate (AITC), can be effectively analyzed in biological samples after derivatization with N-(tert-Butoxycarbonyl)-L-cysteine methyl ester. [] This derivatization step, followed by UHPLC-MS/MS analysis, allows for the simultaneous detection and quantification of AITC and its metabolites. This method has shown promising results in pharmacokinetic studies, highlighting the potential of using derivatization strategies with N-(tert-Butoxycarbonyl)-L-cysteine methyl ester for analyzing similar compounds in complex biological matrices. []
Disclaimer and Information on In-Vitro Research Products
Please be aware that all articles and product information presented on BenchChem are intended solely for informational purposes. The products available for purchase on BenchChem are specifically designed for in-vitro studies, which are conducted outside of living organisms. In-vitro studies, derived from the Latin term "in glass," involve experiments performed in controlled laboratory settings using cells or tissues. It is important to note that these products are not categorized as medicines or drugs, and they have not received approval from the FDA for the prevention, treatment, or cure of any medical condition, ailment, or disease. We must emphasize that any form of bodily introduction of these products into humans or animals is strictly prohibited by law. It is essential to adhere to these guidelines to ensure compliance with legal and ethical standards in research and experimentation.