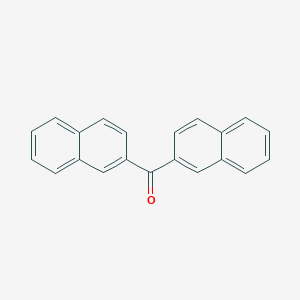
2,2'-Dinaphthyl ketone
Overview
Description
2,2’-Dinaphthyl ketone is a chemical compound with the molecular formula C21H14O . It has a molecular weight of 282.34 . The IUPAC name for this compound is di(2-naphthyl)methanone .
Synthesis Analysis
The synthesis of 2,2’-Dinaphthyl ketone involves several steps . Ketone 19, which is 2,2’-Dinaphthyl ketone, has been prepared by a Grignard reaction of 2-naphthylmagnesium bromide with 2-naphthoyl chloride .
Molecular Structure Analysis
The molecular structure of 2,2’-Dinaphthyl ketone consists of two naphthyl groups attached to a carbonyl group .
Physical And Chemical Properties Analysis
2,2’-Dinaphthyl ketone is a solid at room temperature . It has a density of 1.2±0.1 g/cm3 . The boiling point is 485.7±14.0 °C at 760 mmHg . The compound has a molar refractivity of 91.7±0.3 cm3 .
Scientific Research Applications
Chemical Synthesis
“2,2’-Dinaphthyl ketone” is used in chemical synthesis . It’s a compound with the linear formula C21H14O . The compound is synthesized from di(1-naphthyl)methanol and by a Friedel–Crafts acylation of naphthalene with 2-naphthoyl chloride . It’s also prepared by a Grignard reaction of 2-naphthylmagnesium bromide with 2-naphthoyl chloride .
Conformational Studies
The compound has been used in conformational studies . A systematic DFT study (B3LYP/6-31G(d)) of the conformational spaces of 2,2’-Dinaphthyl ketone and its 1H and 13C NMR chemical shifts has been performed . The study noted a variety of conformations in the crystal structures .
Preparation of Thioketones and Diazomethanes
“2,2’-Dinaphthyl ketone” is used in the preparation of thioketones and diazomethanes . The thioketones are prepared by reactions of the corresponding ketones with Lawesson’s reagent . The diazomethane derivatives are prepared by the HgO oxidation of the respective hydrazones .
Life Science Research
The compound is used in life science research . However, the specific applications in this field are not detailed in the available resources.
Safety and Hazards
properties
IUPAC Name |
dinaphthalen-2-ylmethanone | |
---|---|---|
Source | PubChem | |
URL | https://pubchem.ncbi.nlm.nih.gov | |
Description | Data deposited in or computed by PubChem | |
InChI |
InChI=1S/C21H14O/c22-21(19-11-9-15-5-1-3-7-17(15)13-19)20-12-10-16-6-2-4-8-18(16)14-20/h1-14H | |
Source | PubChem | |
URL | https://pubchem.ncbi.nlm.nih.gov | |
Description | Data deposited in or computed by PubChem | |
InChI Key |
VLEZVYXNVAHDOI-UHFFFAOYSA-N | |
Source | PubChem | |
URL | https://pubchem.ncbi.nlm.nih.gov | |
Description | Data deposited in or computed by PubChem | |
Canonical SMILES |
C1=CC=C2C=C(C=CC2=C1)C(=O)C3=CC4=CC=CC=C4C=C3 | |
Source | PubChem | |
URL | https://pubchem.ncbi.nlm.nih.gov | |
Description | Data deposited in or computed by PubChem | |
Molecular Formula |
C21H14O | |
Source | PubChem | |
URL | https://pubchem.ncbi.nlm.nih.gov | |
Description | Data deposited in or computed by PubChem | |
DSSTOX Substance ID |
DTXSID80449357 | |
Record name | beta-naphthyl ketone | |
Source | EPA DSSTox | |
URL | https://comptox.epa.gov/dashboard/DTXSID80449357 | |
Description | DSSTox provides a high quality public chemistry resource for supporting improved predictive toxicology. | |
Molecular Weight |
282.3 g/mol | |
Source | PubChem | |
URL | https://pubchem.ncbi.nlm.nih.gov | |
Description | Data deposited in or computed by PubChem | |
Product Name |
2,2'-Dinaphthyl ketone | |
CAS RN |
613-56-9 | |
Record name | beta-naphthyl ketone | |
Source | EPA DSSTox | |
URL | https://comptox.epa.gov/dashboard/DTXSID80449357 | |
Description | DSSTox provides a high quality public chemistry resource for supporting improved predictive toxicology. | |
Synthesis routes and methods
Procedure details
Retrosynthesis Analysis
AI-Powered Synthesis Planning: Our tool employs the Template_relevance Pistachio, Template_relevance Bkms_metabolic, Template_relevance Pistachio_ringbreaker, Template_relevance Reaxys, Template_relevance Reaxys_biocatalysis model, leveraging a vast database of chemical reactions to predict feasible synthetic routes.
One-Step Synthesis Focus: Specifically designed for one-step synthesis, it provides concise and direct routes for your target compounds, streamlining the synthesis process.
Accurate Predictions: Utilizing the extensive PISTACHIO, BKMS_METABOLIC, PISTACHIO_RINGBREAKER, REAXYS, REAXYS_BIOCATALYSIS database, our tool offers high-accuracy predictions, reflecting the latest in chemical research and data.
Strategy Settings
Precursor scoring | Relevance Heuristic |
---|---|
Min. plausibility | 0.01 |
Model | Template_relevance |
Template Set | Pistachio/Bkms_metabolic/Pistachio_ringbreaker/Reaxys/Reaxys_biocatalysis |
Top-N result to add to graph | 6 |
Feasible Synthetic Routes
Q & A
Q1: Why is the conformational similarity between 2,2'-dinaphthyl ketone and bis(benzo[b]thiophen-2-yl)methanone relevant?
A1: [] The research highlights that 2,2'-dinaphthyl ketone exhibits significant conformational resemblance to bis(benzo[b]thiophen-2-yl)methanone (also referred to as (I) in the study). This similarity is observed in key structural parameters like interplanar angles, Caryl-Cbridge-Caryl angles, and dihedral angles. This structural resemblance is interesting because it suggests that bis(benzo[b]thiophen-2-yl)methane derivatives could potentially mimic certain properties or activities of compounds containing the dinaphthyl ketone scaffold. Furthermore, the study found comparable crystal packing arrangements between the two compounds. While 2,2'-dinaphthyl ketone exhibits edge-to-face interactions between its naphthyl rings, bis(benzo[b]thiophen-2-yl)methanone displays analogous S...π contacts between its benzo[b]thiophen-2-yl units. This similarity in packing further underscores the potential for these compounds to share similar physical or chemical properties. []
Disclaimer and Information on In-Vitro Research Products
Please be aware that all articles and product information presented on BenchChem are intended solely for informational purposes. The products available for purchase on BenchChem are specifically designed for in-vitro studies, which are conducted outside of living organisms. In-vitro studies, derived from the Latin term "in glass," involve experiments performed in controlled laboratory settings using cells or tissues. It is important to note that these products are not categorized as medicines or drugs, and they have not received approval from the FDA for the prevention, treatment, or cure of any medical condition, ailment, or disease. We must emphasize that any form of bodily introduction of these products into humans or animals is strictly prohibited by law. It is essential to adhere to these guidelines to ensure compliance with legal and ethical standards in research and experimentation.