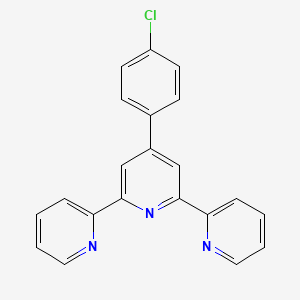
4-(4-chlorophenyl)-2,6-dipyridin-2-ylpyridine
Overview
Description
4’-(4-Chlorophenyl)-2,2’:6’,2’'-terpyridine is a complex organic compound that belongs to the family of terpyridines. This compound is characterized by the presence of a chlorophenyl group attached to the terpyridine core. Terpyridines are known for their ability to form stable complexes with various metal ions, making them valuable in coordination chemistry and materials science.
Preparation Methods
Synthetic Routes and Reaction Conditions
The synthesis of 4’-(4-Chlorophenyl)-2,2’:6’,2’'-terpyridine typically involves the reaction of 4-chlorobenzaldehyde with 2-acetylpyridine in the presence of a base such as potassium carbonate. The reaction proceeds through a condensation mechanism, forming the desired terpyridine compound. The reaction is usually carried out in a solvent like ethanol or methanol under reflux conditions for several hours to ensure complete conversion.
Industrial Production Methods
Industrial production of 4’-(4-Chlorophenyl)-2,2’:6’,2’'-terpyridine follows similar synthetic routes but on a larger scale. The process involves the use of large reactors and optimized reaction conditions to maximize yield and purity. The product is then purified using techniques such as recrystallization or chromatography to obtain the desired compound in high purity.
Chemical Reactions Analysis
Types of Reactions
4’-(4-Chlorophenyl)-2,2’:6’,2’'-terpyridine undergoes various chemical reactions, including:
Oxidation: The compound can be oxidized using oxidizing agents like potassium permanganate or hydrogen peroxide.
Reduction: Reduction reactions can be carried out using reducing agents such as sodium borohydride or lithium aluminum hydride.
Substitution: The chlorophenyl group can undergo nucleophilic substitution reactions with nucleophiles like amines or thiols.
Common Reagents and Conditions
Oxidation: Potassium permanganate in an acidic medium.
Reduction: Sodium borohydride in methanol.
Substitution: Ammonia or primary amines in ethanol.
Major Products Formed
Oxidation: Formation of corresponding carboxylic acids or ketones.
Reduction: Formation of alcohols or amines.
Substitution: Formation of substituted terpyridine derivatives.
Scientific Research Applications
4’-(4-Chlorophenyl)-2,2’:6’,2’'-terpyridine has a wide range of applications in scientific research:
Chemistry: Used as a ligand in coordination chemistry to form metal complexes with unique properties.
Biology: Investigated for its potential as a fluorescent probe for biological imaging.
Medicine: Explored for its potential as an anticancer agent due to its ability to interact with DNA.
Industry: Utilized in the development of advanced materials such as organic semiconductors and light-emitting diodes (LEDs).
Mechanism of Action
The mechanism of action of 4’-(4-Chlorophenyl)-2,2’:6’,2’'-terpyridine involves its ability to form stable complexes with metal ions. These complexes can interact with biological molecules such as DNA, leading to potential therapeutic effects. The compound can also act as a photosensitizer, generating reactive oxygen species upon exposure to light, which can induce cell death in cancer cells.
Comparison with Similar Compounds
Similar Compounds
- 4’-(4-Bromophenyl)-2,2’:6’,2’'-terpyridine
- 4’-(4-Methylphenyl)-2,2’:6’,2’'-terpyridine
- 4’-(4-Nitrophenyl)-2,2’:6’,2’'-terpyridine
Uniqueness
4’-(4-Chlorophenyl)-2,2’:6’,2’'-terpyridine is unique due to the presence of the chlorophenyl group, which imparts distinct electronic and steric properties. This makes it particularly suitable for forming stable metal complexes with specific properties, making it valuable in various applications such as catalysis, materials science, and medicinal chemistry.
Properties
IUPAC Name |
4-(4-chlorophenyl)-2,6-dipyridin-2-ylpyridine | |
---|---|---|
Source | PubChem | |
URL | https://pubchem.ncbi.nlm.nih.gov | |
Description | Data deposited in or computed by PubChem | |
InChI |
InChI=1S/C21H14ClN3/c22-17-9-7-15(8-10-17)16-13-20(18-5-1-3-11-23-18)25-21(14-16)19-6-2-4-12-24-19/h1-14H | |
Source | PubChem | |
URL | https://pubchem.ncbi.nlm.nih.gov | |
Description | Data deposited in or computed by PubChem | |
InChI Key |
UPOMZZQMFGCHGB-UHFFFAOYSA-N | |
Source | PubChem | |
URL | https://pubchem.ncbi.nlm.nih.gov | |
Description | Data deposited in or computed by PubChem | |
Canonical SMILES |
C1=CC=NC(=C1)C2=CC(=CC(=N2)C3=CC=CC=N3)C4=CC=C(C=C4)Cl | |
Source | PubChem | |
URL | https://pubchem.ncbi.nlm.nih.gov | |
Description | Data deposited in or computed by PubChem | |
Molecular Formula |
C21H14ClN3 | |
Source | PubChem | |
URL | https://pubchem.ncbi.nlm.nih.gov | |
Description | Data deposited in or computed by PubChem | |
DSSTOX Substance ID |
DTXSID60446622 | |
Record name | 2~4~-(4-Chlorophenyl)-1~2~,2~2~:2~6~,3~2~-terpyridine | |
Source | EPA DSSTox | |
URL | https://comptox.epa.gov/dashboard/DTXSID60446622 | |
Description | DSSTox provides a high quality public chemistry resource for supporting improved predictive toxicology. | |
Molecular Weight |
343.8 g/mol | |
Source | PubChem | |
URL | https://pubchem.ncbi.nlm.nih.gov | |
Description | Data deposited in or computed by PubChem | |
CAS No. |
89972-75-8 | |
Record name | 2~4~-(4-Chlorophenyl)-1~2~,2~2~:2~6~,3~2~-terpyridine | |
Source | EPA DSSTox | |
URL | https://comptox.epa.gov/dashboard/DTXSID60446622 | |
Description | DSSTox provides a high quality public chemistry resource for supporting improved predictive toxicology. | |
Record name | 4'-(4-Chlorophenyl)-2,2':6',2"-terpyridine 200000042041 | |
Source | European Chemicals Agency (ECHA) | |
URL | https://echa.europa.eu/information-on-chemicals | |
Description | The European Chemicals Agency (ECHA) is an agency of the European Union which is the driving force among regulatory authorities in implementing the EU's groundbreaking chemicals legislation for the benefit of human health and the environment as well as for innovation and competitiveness. | |
Explanation | Use of the information, documents and data from the ECHA website is subject to the terms and conditions of this Legal Notice, and subject to other binding limitations provided for under applicable law, the information, documents and data made available on the ECHA website may be reproduced, distributed and/or used, totally or in part, for non-commercial purposes provided that ECHA is acknowledged as the source: "Source: European Chemicals Agency, http://echa.europa.eu/". Such acknowledgement must be included in each copy of the material. ECHA permits and encourages organisations and individuals to create links to the ECHA website under the following cumulative conditions: Links can only be made to webpages that provide a link to the Legal Notice page. | |
Retrosynthesis Analysis
AI-Powered Synthesis Planning: Our tool employs the Template_relevance Pistachio, Template_relevance Bkms_metabolic, Template_relevance Pistachio_ringbreaker, Template_relevance Reaxys, Template_relevance Reaxys_biocatalysis model, leveraging a vast database of chemical reactions to predict feasible synthetic routes.
One-Step Synthesis Focus: Specifically designed for one-step synthesis, it provides concise and direct routes for your target compounds, streamlining the synthesis process.
Accurate Predictions: Utilizing the extensive PISTACHIO, BKMS_METABOLIC, PISTACHIO_RINGBREAKER, REAXYS, REAXYS_BIOCATALYSIS database, our tool offers high-accuracy predictions, reflecting the latest in chemical research and data.
Strategy Settings
Precursor scoring | Relevance Heuristic |
---|---|
Min. plausibility | 0.01 |
Model | Template_relevance |
Template Set | Pistachio/Bkms_metabolic/Pistachio_ringbreaker/Reaxys/Reaxys_biocatalysis |
Top-N result to add to graph | 6 |
Feasible Synthetic Routes
Disclaimer and Information on In-Vitro Research Products
Please be aware that all articles and product information presented on BenchChem are intended solely for informational purposes. The products available for purchase on BenchChem are specifically designed for in-vitro studies, which are conducted outside of living organisms. In-vitro studies, derived from the Latin term "in glass," involve experiments performed in controlled laboratory settings using cells or tissues. It is important to note that these products are not categorized as medicines or drugs, and they have not received approval from the FDA for the prevention, treatment, or cure of any medical condition, ailment, or disease. We must emphasize that any form of bodily introduction of these products into humans or animals is strictly prohibited by law. It is essential to adhere to these guidelines to ensure compliance with legal and ethical standards in research and experimentation.