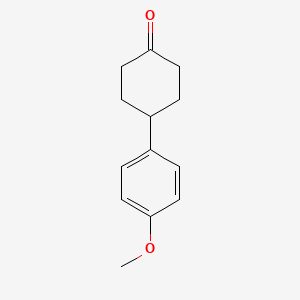
4-(4-Methoxyphenyl)cyclohexanone
Overview
Description
4-(4-Methoxyphenyl)cyclohexanone is a cyclohexanone derivative featuring a methoxy-substituted phenyl group at the 4-position of the cyclohexanone ring. Its molecular formula is C₁₃H₁₆O₂, with a molecular weight of 204.26 g/mol. This compound is widely used in organic synthesis, particularly in the preparation of pharmaceuticals such as selective estrogen receptor β agonists () and the antidepressant venlafaxine (). Its synthesis typically involves alkylation or condensation reactions with precursors like 4-methoxyphenyl acetonitrile and cyclohexanone under basic conditions ().
The methoxy group enhances lipophilicity and electronic stability, making it advantageous in drug design for improved bioavailability and receptor binding ().
Preparation Methods
Oxidation of 4-Methoxycyclohexanol Using Hydrogen Peroxide and Phosphotungstic Acid Catalyst
One of the most well-documented and efficient methods for synthesizing 4-(4-methoxyphenyl)cyclohexanone involves the oxidation of 4-methoxycyclohexanol using hydrogen peroxide as the oxidizing agent, catalyzed by a phosphotungstic acid molecular sieve catalyst in a tubular reactor system.
Process Overview
- Raw Materials : 4-methoxycyclohexanol (30–50% solution) and hydrogen peroxide (30–50% solution).
- Catalyst : Phosphotungstic acid supported on 5A molecular sieve, prepared by refluxing phosphotungstic acid with molecular sieve in dehydrated alcohol, followed by drying and roasting at 200 °C.
- Reactor : Tubular glass reactor (20 mm internal diameter, 60 cm length), vertically fixed with temperature control.
- Reaction Conditions : Temperature maintained between 70–90 °C.
- Feed Ratios : Molar ratio of 4-methoxycyclohexanol to hydrogen peroxide ranges from 1:1.05 to 1:1.5.
- Feed Rates : Alcohol solution feed rate between 5–15 g/min; hydrogen peroxide feed rate adjusted accordingly for simultaneous addition.
- Reaction Time : Varies from 35 minutes to 2.9 hours depending on conditions.
Extraction and Purification
- Post-reaction, the aqueous solution containing 4-methoxycyclohexanone is extracted using organic solvents such as toluene, ethylene dichloride, ethyl acetate, or methyl tert-butyl ether.
- The organic phase is collected, and the product is precipitated to obtain high-purity 4-methoxycyclohexanone.
Catalyst Preparation
- Phosphotungstic acid and 5A molecular sieve are mixed in a 1:1 mass ratio.
- The mixture is refluxed in dehydrated alcohol for 2 hours, cooled, filtered, dried, and roasted at 200 °C for 4 hours to obtain the active catalyst.
Alternative Catalytic Reduction Approaches (Contextual Reference)
While the direct oxidation method is predominant for this compound, related compounds such as 4-(4-hydroxyphenyl)cyclohexanol have been prepared using catalytic hydrogenation methods involving palladium, platinum, or Raney nickel catalysts under hydrogen atmosphere. These methods typically reduce aromatic ketones or phenols to the corresponding cyclohexanol derivatives but are less directly applicable to the ketone synthesis of this compound itself.
Summary Table of Preparation Method
Step | Details |
---|---|
Starting Material | 4-Methoxycyclohexanol (30–50% solution) |
Oxidizing Agent | Hydrogen peroxide (30–50% solution) |
Catalyst | Phosphotungstic acid on 5A molecular sieve (1:1 mass ratio) |
Reactor | Tubular glass reactor (20 mm diameter, 60 cm length), vertical, temperature controlled |
Reaction Temperature | 70–90 °C |
Feed Ratio (Alcohol:H2O2) | 1:1.05 to 1:1.5 (molar) |
Feed Rate | Alcohol: 5–15 g/min; H2O2 adjusted simultaneously |
Reaction Time | 35 minutes to 2.9 hours |
Extraction Solvent | Toluene, ethylene dichloride, ethyl acetate, or methyl tert-butyl ether |
Product Purity | 98.5–98.9% (by GC analysis) |
Yield | 98.3–98.8% |
Chemical Reactions Analysis
Types of Reactions
4-(4-Methoxyphenyl)cyclohexanone undergoes various chemical reactions, including:
Oxidation: The compound can be oxidized to form corresponding carboxylic acids or ketones.
Reduction: Reduction reactions can convert the ketone group to an alcohol.
Substitution: The methoxy group can be substituted with other functional groups under appropriate conditions.
Common Reagents and Conditions
Oxidation: Common oxidizing agents include potassium permanganate and chromium trioxide.
Reduction: Sodium borohydride or lithium aluminum hydride are often used as reducing agents.
Substitution: Halogenating agents like bromine or chlorine can be used for substitution reactions.
Major Products Formed
Oxidation: Formation of carboxylic acids or diketones.
Reduction: Formation of alcohols.
Substitution: Formation of halogenated derivatives.
Scientific Research Applications
Pharmaceutical Synthesis
One of the primary applications of 4-(4-Methoxyphenyl)cyclohexanone is in the synthesis of antidepressants such as Venlafaxine. The compound is utilized as an intermediate in the preparation of 1-[2-dimethylamino-(4-methoxyphenyl)ethyl]cyclohexanol, which is known for its efficacy in treating major depressive disorder and anxiety disorders. The process involves treating 4-methoxyphenyl acetonitrile with cyclohexanone in the presence of alkali hydroxide to yield intermediates that are further processed into active pharmaceutical ingredients (APIs) .
Anticancer Research
Recent studies have highlighted the potential anticancer properties of cyclohexanone derivatives, including those containing the 4-(4-Methoxyphenyl) moiety. Functionalized cyclohexanones are being investigated for their ability to inhibit cancer cell proliferation and induce apoptosis. For instance, a library of malonamide-based compounds derived from cyclohexanones demonstrated significant anticancer activity . The structural framework provided by this compound enhances bioactivity through specific interactions with biological targets.
Designer Drug Development
Research has also explored derivatives of this compound in the context of designer drugs. Compounds such as N-(4-methoxyphenyl)piperazine have been synthesized and studied for their psychoactive properties, which may mimic those of traditional stimulants while exhibiting lower abuse potential . This area of research is crucial for understanding the pharmacological profiles and safety profiles of new psychoactive substances.
Case Study 1: Venlafaxine Synthesis
A patent describes an efficient method for synthesizing Venlafaxine using this compound as a key intermediate. The process emphasizes high yield and purity, making it suitable for industrial applications .
Step | Description |
---|---|
1 | Treating 4-methoxyphenyl acetonitrile with cyclohexanone in alkaline conditions |
2 | Reduction of intermediates to produce Venlafaxine hydrochloride |
Case Study 2: Anticancer Activity
A study conducted on various functionalized cyclohexanones revealed that derivatives containing the methoxyphenyl group exhibited potent inhibitory effects against cancer cell lines. The research utilized in vitro assays to evaluate cell viability and apoptosis induction .
Compound | IC50 (µM) | Mechanism |
---|---|---|
Compound A | 15 | Apoptosis induction |
Compound B | 25 | Cell cycle arrest |
Mechanism of Action
The mechanism of action of 4-(4-Methoxyphenyl)cyclohexanone involves its interaction with specific molecular targets. For instance, it can act as a ligand for the glutamate NMDA receptor, similar to other methoxy analogues of phencyclidine. This interaction can influence neurotransmitter systems and potentially exhibit psychotomimetic effects .
Comparison with Similar Compounds
Comparison with Structural Analogs
Structural and Functional Group Variations
The following table highlights key structural analogs and their distinguishing features:
Key Observations:
- Electronic Effects: The methoxy group in this compound donates electron density via resonance, enhancing stability compared to the electron-withdrawing cyano group in 4-Cyano-4-(4-fluorophenyl)cyclohexanone ().
- Sulfonyl Derivatives: The sulfonyl group in 4-(4-Chlorophenylsulfonyl)-4-(2,5-difluorophenyl)cyclohexanone introduces steric bulk and polarizability, often utilized in protease inhibitors or kinase-targeted drugs ().
Catalytic Hydrogenation
Cyclohexanone derivatives are frequently hydrogenated to cyclohexanol intermediates. For example, this compound can be reduced to 1-[cyano(4-methoxyphenyl)methyl]cyclohexanol, a precursor to venlafaxine (). In contrast, 4-Hydroxy-4-methylcyclohexanone undergoes slower hydrogenation due to steric hindrance from the methyl group ().
Enzymatic Oxidation
Cyclohexanone monooxygenase (CHMO) catalyzes the Baeyer-Villiger oxidation of cyclohexanones to lactones.
Biological Activity
4-(4-Methoxyphenyl)cyclohexanone, also known as a substituted cyclohexanone, has garnered attention in pharmacological research due to its potential biological activities. This article explores its biological properties, including its effects on various biological systems, mechanisms of action, and relevant case studies.
Chemical Structure and Properties
The compound this compound features a cyclohexanone ring substituted with a para-methoxyphenyl group. Its molecular formula is , and it exhibits both lipophilic and hydrophilic characteristics, which may influence its interaction with biological targets.
1. Antioxidant Activity
Research indicates that this compound possesses significant antioxidant properties. A study demonstrated that compounds with similar structures showed effective free radical scavenging activity, which is crucial for mitigating oxidative stress in cells .
2. Anti-inflammatory Effects
The compound has been evaluated for its anti-inflammatory effects, particularly through the inhibition of pro-inflammatory cytokines and enzymes. In vitro assays revealed that it could inhibit lipoxygenase (LOX) and cyclooxygenase (COX) activities, leading to reduced production of inflammatory mediators such as prostaglandins and leukotrienes .
3. Antimicrobial Activity
Preliminary studies have shown that this compound exhibits antimicrobial activity against various bacterial strains. The compound's effectiveness was assessed using the disc diffusion method, revealing zones of inhibition comparable to standard antibiotics .
The biological activities of this compound can be attributed to several mechanisms:
- Antioxidant Mechanism : The methoxy group enhances electron donation, allowing the compound to neutralize free radicals effectively.
- Enzyme Inhibition : By interacting with the active sites of LOX and COX enzymes, the compound can prevent the conversion of arachidonic acid into inflammatory mediators.
- Membrane Disruption : The lipophilic nature of the cyclohexanone structure may allow it to integrate into bacterial membranes, disrupting their integrity and function.
Case Study 1: Antioxidant Efficacy
In a controlled study assessing various phenolic compounds for their antioxidant capacity, this compound demonstrated an IC50 value of approximately 50 µM, indicating potent activity compared to other tested compounds .
Case Study 2: Anti-inflammatory Potential
A study investigating the anti-inflammatory properties of this compound involved treating human neutrophils with varying concentrations. Results showed a dose-dependent reduction in IL-6 and TNF-alpha levels, suggesting potential therapeutic applications in inflammatory diseases .
Data Tables
Biological Activity | Assay Type | IC50 Value (µM) |
---|---|---|
Antioxidant | DPPH Scavenging | 50 |
Anti-inflammatory (IL-6) | Neutrophil Culture | 30 |
Antimicrobial (E. coli) | Disc Diffusion | Zone: 15 mm |
Q & A
Q. Basic: What are the common synthetic routes for 4-(4-Methoxyphenyl)cyclohexanone?
A key method involves condensation reactions between 4-methoxyphenyl acetonitrile and cyclohexanone in the presence of alkali hydroxide and a superbase, followed by reduction steps to yield intermediates . Another approach includes oxime formation via hydroxylamine hydrochloride treatment under acidic conditions, as demonstrated in structurally similar cyclohexanone derivatives . These routes emphasize the importance of ketone reactivity and functional group compatibility in synthesis design.
Q. Basic: How is the structure of this compound characterized in research settings?
Structural characterization relies on spectroscopic techniques :
- NMR spectroscopy (¹H and ¹³C) identifies proton environments and carbon frameworks, particularly distinguishing the methoxy group and cyclohexanone ring .
- IR spectroscopy detects key functional groups (e.g., C=O stretch at ~1700 cm⁻¹) and hydrogen bonding in tautomeric forms .
- HRMS (High-Resolution Mass Spectrometry) confirms molecular weight and fragmentation patterns for purity assessment .
Q. Advanced: How does the methoxy substituent influence the reactivity of this compound in nucleophilic additions?
The methoxy group exerts electronic and steric effects :
- Electron-donating resonance increases electron density on the aromatic ring, potentially directing electrophilic attacks to specific positions .
- Steric hindrance from the methoxy group may slow nucleophilic additions to the cyclohexanone carbonyl, requiring optimized reaction conditions (e.g., catalysts or elevated temperatures) . Computational studies (e.g., DFT) can further elucidate substituent effects on transition states .
Q. Advanced: What strategies resolve contradictions in biological activity data for derivatives of this compound?
Discrepancies in biological activity (e.g., antidepressant vs. anti-tubercular effects ) may arise from:
- Structural variations : Substituent position (e.g., para-methoxy vs. ortho) alters binding affinity to targets like serotonin transporters or mycobacterial enzymes .
- Assay conditions : Differences in cell lines, concentrations, or pharmacokinetic parameters (e.g., solubility) require standardization .
- Mechanistic studies : Comparative dose-response curves and receptor-binding assays help validate target specificity .
Q. Basic: What are the key physical and chemical properties of this compound relevant to experimental design?
Critical properties include:
- Solubility : High solubility in polar solvents (e.g., ethanol, methanol) facilitates reactions in homogeneous phases .
- Stability : Sensitivity to strong acids/bases necessitates pH-controlled environments .
- Melting Point : ~200°C (similar to analogs) aids in purification via recrystallization .
- Reactivity : Susceptibility to oxidation/reduction mandates inert atmospheres for ketone preservation .
Q. Advanced: How can computational modeling predict interactions of this compound derivatives with biological targets?
Molecular docking and MD (Molecular Dynamics) simulations are used to:
- Map binding pockets (e.g., estrogen receptor-α) by analyzing hydrogen bonding and hydrophobic interactions with the methoxyphenyl moiety .
- Predict ADMET (Absorption, Distribution, Metabolism, Excretion, Toxicity) profiles via QSAR (Quantitative Structure-Activity Relationship) models, optimizing lead compounds .
- Validate hypotheses from conflicting experimental data, such as contrasting IC₅₀ values in enzyme inhibition assays .
Q. Basic: What safety precautions are essential when handling this compound?
- PPE (Personal Protective Equipment) : Gloves, lab coats, and goggles to prevent skin/eye contact .
- Ventilation : Use fume hoods to avoid inhalation of vapors .
- Spill Management : Absorb with inert materials (e.g., silica gel) and dispose as hazardous waste .
- Emergency Protocols : Immediate rinsing for eye/skin exposure and medical consultation .
Q. Advanced: How is this compound utilized in enantioselective synthesis?
As a precursor, it enables diastereoselective fluorodesilylation for synthesizing vitamin D₃ analogs:
- The cyclohexanone ring serves as a scaffold for introducing chiral centers via substrate-controlled reactions .
- Silyl ether intermediates (e.g., tert-butyldimethylsilyloxy derivatives) enhance stereochemical control in fluorination steps .
Q. Advanced: What role does tautomerism play in the reactivity of this compound derivatives?
- Keto-enol tautomerism in cyclohexanone derivatives influences hydrogen bonding and stability:
- Enol forms participate in Michael additions or cyclization reactions , expanding synthetic utility .
- Tautomeric equilibria are detectable via IR broad peaks (~3400 cm⁻¹) and NMR exchange signals .
Q. Basic: What analytical techniques quantify this compound in complex mixtures?
- HPLC/UPLC with UV detection (λ ~270 nm) separates and quantifies the compound alongside impurities .
- GC-MS is suitable for volatile derivatives, leveraging ketone fragmentation patterns for identification .
- Titration methods (e.g., hydroxylamine hydrochloride for ketone quantification) provide complementary data .
Properties
IUPAC Name |
4-(4-methoxyphenyl)cyclohexan-1-one | |
---|---|---|
Source | PubChem | |
URL | https://pubchem.ncbi.nlm.nih.gov | |
Description | Data deposited in or computed by PubChem | |
InChI |
InChI=1S/C13H16O2/c1-15-13-8-4-11(5-9-13)10-2-6-12(14)7-3-10/h4-5,8-10H,2-3,6-7H2,1H3 | |
Source | PubChem | |
URL | https://pubchem.ncbi.nlm.nih.gov | |
Description | Data deposited in or computed by PubChem | |
InChI Key |
ZMMQLFXRGHSTSW-UHFFFAOYSA-N | |
Source | PubChem | |
URL | https://pubchem.ncbi.nlm.nih.gov | |
Description | Data deposited in or computed by PubChem | |
Canonical SMILES |
COC1=CC=C(C=C1)C2CCC(=O)CC2 | |
Source | PubChem | |
URL | https://pubchem.ncbi.nlm.nih.gov | |
Description | Data deposited in or computed by PubChem | |
Molecular Formula |
C13H16O2 | |
Source | PubChem | |
URL | https://pubchem.ncbi.nlm.nih.gov | |
Description | Data deposited in or computed by PubChem | |
DSSTOX Substance ID |
DTXSID10450451 | |
Record name | 4-(4-methoxyphenyl)cyclohexanone | |
Source | EPA DSSTox | |
URL | https://comptox.epa.gov/dashboard/DTXSID10450451 | |
Description | DSSTox provides a high quality public chemistry resource for supporting improved predictive toxicology. | |
Molecular Weight |
204.26 g/mol | |
Source | PubChem | |
URL | https://pubchem.ncbi.nlm.nih.gov | |
Description | Data deposited in or computed by PubChem | |
CAS No. |
5309-16-0 | |
Record name | 4-(4-Methoxyphenyl)cyclohexanone | |
Source | CAS Common Chemistry | |
URL | https://commonchemistry.cas.org/detail?cas_rn=5309-16-0 | |
Description | CAS Common Chemistry is an open community resource for accessing chemical information. Nearly 500,000 chemical substances from CAS REGISTRY cover areas of community interest, including common and frequently regulated chemicals, and those relevant to high school and undergraduate chemistry classes. This chemical information, curated by our expert scientists, is provided in alignment with our mission as a division of the American Chemical Society. | |
Explanation | The data from CAS Common Chemistry is provided under a CC-BY-NC 4.0 license, unless otherwise stated. | |
Record name | 4-(4-Methoxyphenyl)cyclohexanone | |
Source | ChemIDplus | |
URL | https://pubchem.ncbi.nlm.nih.gov/substance/?source=chemidplus&sourceid=0005309160 | |
Description | ChemIDplus is a free, web search system that provides access to the structure and nomenclature authority files used for the identification of chemical substances cited in National Library of Medicine (NLM) databases, including the TOXNET system. | |
Record name | 4-(4-methoxyphenyl)cyclohexanone | |
Source | EPA DSSTox | |
URL | https://comptox.epa.gov/dashboard/DTXSID10450451 | |
Description | DSSTox provides a high quality public chemistry resource for supporting improved predictive toxicology. | |
Record name | 4-(4-methoxyphenyl)cyclohexanone | |
Source | European Chemicals Agency (ECHA) | |
URL | https://echa.europa.eu/substance-information/-/substanceinfo/100.123.772 | |
Description | The European Chemicals Agency (ECHA) is an agency of the European Union which is the driving force among regulatory authorities in implementing the EU's groundbreaking chemicals legislation for the benefit of human health and the environment as well as for innovation and competitiveness. | |
Explanation | Use of the information, documents and data from the ECHA website is subject to the terms and conditions of this Legal Notice, and subject to other binding limitations provided for under applicable law, the information, documents and data made available on the ECHA website may be reproduced, distributed and/or used, totally or in part, for non-commercial purposes provided that ECHA is acknowledged as the source: "Source: European Chemicals Agency, http://echa.europa.eu/". Such acknowledgement must be included in each copy of the material. ECHA permits and encourages organisations and individuals to create links to the ECHA website under the following cumulative conditions: Links can only be made to webpages that provide a link to the Legal Notice page. | |
Synthesis routes and methods I
Procedure details
Synthesis routes and methods II
Procedure details
Synthesis routes and methods III
Procedure details
Synthesis routes and methods IV
Procedure details
Retrosynthesis Analysis
AI-Powered Synthesis Planning: Our tool employs the Template_relevance Pistachio, Template_relevance Bkms_metabolic, Template_relevance Pistachio_ringbreaker, Template_relevance Reaxys, Template_relevance Reaxys_biocatalysis model, leveraging a vast database of chemical reactions to predict feasible synthetic routes.
One-Step Synthesis Focus: Specifically designed for one-step synthesis, it provides concise and direct routes for your target compounds, streamlining the synthesis process.
Accurate Predictions: Utilizing the extensive PISTACHIO, BKMS_METABOLIC, PISTACHIO_RINGBREAKER, REAXYS, REAXYS_BIOCATALYSIS database, our tool offers high-accuracy predictions, reflecting the latest in chemical research and data.
Strategy Settings
Precursor scoring | Relevance Heuristic |
---|---|
Min. plausibility | 0.01 |
Model | Template_relevance |
Template Set | Pistachio/Bkms_metabolic/Pistachio_ringbreaker/Reaxys/Reaxys_biocatalysis |
Top-N result to add to graph | 6 |
Feasible Synthetic Routes
Disclaimer and Information on In-Vitro Research Products
Please be aware that all articles and product information presented on BenchChem are intended solely for informational purposes. The products available for purchase on BenchChem are specifically designed for in-vitro studies, which are conducted outside of living organisms. In-vitro studies, derived from the Latin term "in glass," involve experiments performed in controlled laboratory settings using cells or tissues. It is important to note that these products are not categorized as medicines or drugs, and they have not received approval from the FDA for the prevention, treatment, or cure of any medical condition, ailment, or disease. We must emphasize that any form of bodily introduction of these products into humans or animals is strictly prohibited by law. It is essential to adhere to these guidelines to ensure compliance with legal and ethical standards in research and experimentation.