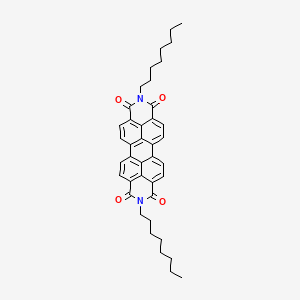
Ptcdi-C8
Overview
Description
N,N′-Dioctyl-3,4,9,10-perylenedicarboximide, commonly known as PTCDI-C8, is an organic semiconductor that belongs to the family of perylene diimides. This compound is renowned for its high electron mobility and stability, making it a valuable material in the field of organic electronics. This compound is particularly noted for its applications in optoelectronic devices such as organic field-effect transistors, light-emitting diodes, and photovoltaic cells .
Mechanism of Action
Target of Action
N,N′-Dioctyl-3,4,9,10 perylenedicarboximide (PTCDI-C8) is an organic semiconductor that primarily targets optoelectronic devices . It is used in the fabrication of a wide range of devices such as solar cells, thermoelectric generators, thin film photovoltaics, and field-effect transistors . The role of this compound in these devices is based on its spectral and optical properties .
Mode of Action
The interaction of this compound with its targets involves the manipulation of its optical properties. The spectral parameters such as molar/mass extinction coefficients and absorption coefficient of the this compound molecule are calculated to determine its optical properties . These properties, including angle of incidence/refraction, optical band gap, real and imaginary parts of dielectric constant, loss factor, and electrical susceptibility, are crucial for its function in optoelectronic devices .
Biochemical Pathways
The biochemical pathways affected by this compound are primarily related to the transmission of light and electricity. The absorption bands of this compound semiconductor exhibit a vibronic structure with seven peaks at specific energy levels . These peaks influence the spectral parameters and, consequently, the optical properties of this compound, affecting the downstream effects in optoelectronic devices .
Result of Action
The result of this compound’s action is the enhancement of the performance of optoelectronic devices. For instance, organic field-effect transistors (OFETs) fabricated with this compound have shown a high mobility, which is better than the performance previously reported for this compound based devices . Moreover, these devices exhibit robust air stability and remain stable after exposure in air over 12 days .
Action Environment
The action of this compound can be influenced by environmental factors such as the solvent used and the annealing time during the fabrication process . These factors greatly influence the formation of organic wires, which are crucial for the performance of optoelectronic devices . Therefore, careful control of the action environment is essential for maximizing the efficacy and stability of this compound in its applications.
Preparation Methods
The synthesis of PTCDI-C8 typically involves the reaction of perylene-3,4,9,10-tetracarboxylic dianhydride with octylamine. The reaction is carried out under reflux conditions in a suitable solvent such as quinoline. The resulting product is then purified through recrystallization or chromatography to obtain high-purity this compound .
Industrial production methods for this compound often involve large-scale synthesis using similar reaction conditions but optimized for higher yields and purity. Solvent phase-transfer and solvent-induced precipitation are common techniques used to produce high-quality crystalline nanowires of this compound .
Chemical Reactions Analysis
PTCDI-C8 undergoes various chemical reactions, including:
Oxidation: this compound can be oxidized using strong oxidizing agents, leading to the formation of perylene diimide derivatives with different functional groups.
Reduction: Reduction of this compound can be achieved using reducing agents such as sodium borohydride, resulting in the formation of reduced perylene diimide compounds.
Substitution: this compound can undergo substitution reactions where the octyl groups are replaced with other alkyl or functional groups.
Common reagents used in these reactions include oxidizing agents like potassium permanganate, reducing agents like sodium borohydride, and nucleophiles such as alkyl halides. The major products formed from these reactions are various derivatives of perylene diimide with altered electronic and optical properties .
Scientific Research Applications
PTCDI-C8 has a wide range of scientific research applications, including:
Chemistry: this compound is used as a building block for the synthesis of more complex organic semiconductors. Its high electron mobility makes it an ideal candidate for studying charge transport mechanisms in organic materials.
Biology: this compound has been explored for use in biosensors due to its ability to interact with biological molecules and its stable electronic properties.
Medicine: Research is ongoing into the potential use of this compound in medical imaging and drug delivery systems, leveraging its optoelectronic properties.
Industry: this compound is widely used in the fabrication of organic field-effect transistors, light-emitting diodes, and photovoltaic cells. .
Comparison with Similar Compounds
PTCDI-C8 is part of a broader family of perylene diimides, which includes compounds such as N,N′-dialkyl-3,4,9,10-perylenetetracarboxylic diimides (PTCDI-Cn). Compared to other similar compounds, this compound is unique due to its high electron mobility and stability under various environmental conditions. Other similar compounds include:
PTCDI-C5: N,N′-dihexyl-3,4,9,10-perylenetetracarboxylic diimide
PTCDI-C10: N,N′-didecyl-3,4,9,10-perylenetetracarboxylic diimide
PTCDI-BP2C10: N,N′-bis(2-phenylethyl)-3,4,9,10-perylenetetracarboxylic diimide
These compounds share similar core structures but differ in their alkyl chain lengths and substituents, which influence their electronic and optical properties .
Properties
IUPAC Name |
7,18-dioctyl-7,18-diazaheptacyclo[14.6.2.22,5.03,12.04,9.013,23.020,24]hexacosa-1(23),2,4,9,11,13,15,20(24),21,25-decaene-6,8,17,19-tetrone | |
---|---|---|
Source | PubChem | |
URL | https://pubchem.ncbi.nlm.nih.gov | |
Description | Data deposited in or computed by PubChem | |
InChI |
InChI=1S/C40H42N2O4/c1-3-5-7-9-11-13-23-41-37(43)29-19-15-25-27-17-21-31-36-32(40(46)42(39(31)45)24-14-12-10-8-6-4-2)22-18-28(34(27)36)26-16-20-30(38(41)44)35(29)33(25)26/h15-22H,3-14,23-24H2,1-2H3 | |
Source | PubChem | |
URL | https://pubchem.ncbi.nlm.nih.gov | |
Description | Data deposited in or computed by PubChem | |
InChI Key |
YFGMQDNQVFJKTR-UHFFFAOYSA-N | |
Source | PubChem | |
URL | https://pubchem.ncbi.nlm.nih.gov | |
Description | Data deposited in or computed by PubChem | |
Canonical SMILES |
CCCCCCCCN1C(=O)C2=C3C(=CC=C4C3=C(C=C2)C5=C6C4=CC=C7C6=C(C=C5)C(=O)N(C7=O)CCCCCCCC)C1=O | |
Source | PubChem | |
URL | https://pubchem.ncbi.nlm.nih.gov | |
Description | Data deposited in or computed by PubChem | |
Molecular Formula |
C40H42N2O4 | |
Source | PubChem | |
URL | https://pubchem.ncbi.nlm.nih.gov | |
Description | Data deposited in or computed by PubChem | |
DSSTOX Substance ID |
DTXSID80443916 | |
Record name | PTCDI-C8 | |
Source | EPA DSSTox | |
URL | https://comptox.epa.gov/dashboard/DTXSID80443916 | |
Description | DSSTox provides a high quality public chemistry resource for supporting improved predictive toxicology. | |
Molecular Weight |
614.8 g/mol | |
Source | PubChem | |
URL | https://pubchem.ncbi.nlm.nih.gov | |
Description | Data deposited in or computed by PubChem | |
CAS No. |
78151-58-3 | |
Record name | PTCDI-C8 | |
Source | EPA DSSTox | |
URL | https://comptox.epa.gov/dashboard/DTXSID80443916 | |
Description | DSSTox provides a high quality public chemistry resource for supporting improved predictive toxicology. | |
Record name | 78151-58-3 | |
Source | European Chemicals Agency (ECHA) | |
URL | https://echa.europa.eu/information-on-chemicals | |
Description | The European Chemicals Agency (ECHA) is an agency of the European Union which is the driving force among regulatory authorities in implementing the EU's groundbreaking chemicals legislation for the benefit of human health and the environment as well as for innovation and competitiveness. | |
Explanation | Use of the information, documents and data from the ECHA website is subject to the terms and conditions of this Legal Notice, and subject to other binding limitations provided for under applicable law, the information, documents and data made available on the ECHA website may be reproduced, distributed and/or used, totally or in part, for non-commercial purposes provided that ECHA is acknowledged as the source: "Source: European Chemicals Agency, http://echa.europa.eu/". Such acknowledgement must be included in each copy of the material. ECHA permits and encourages organisations and individuals to create links to the ECHA website under the following cumulative conditions: Links can only be made to webpages that provide a link to the Legal Notice page. | |
Retrosynthesis Analysis
AI-Powered Synthesis Planning: Our tool employs the Template_relevance Pistachio, Template_relevance Bkms_metabolic, Template_relevance Pistachio_ringbreaker, Template_relevance Reaxys, Template_relevance Reaxys_biocatalysis model, leveraging a vast database of chemical reactions to predict feasible synthetic routes.
One-Step Synthesis Focus: Specifically designed for one-step synthesis, it provides concise and direct routes for your target compounds, streamlining the synthesis process.
Accurate Predictions: Utilizing the extensive PISTACHIO, BKMS_METABOLIC, PISTACHIO_RINGBREAKER, REAXYS, REAXYS_BIOCATALYSIS database, our tool offers high-accuracy predictions, reflecting the latest in chemical research and data.
Strategy Settings
Precursor scoring | Relevance Heuristic |
---|---|
Min. plausibility | 0.01 |
Model | Template_relevance |
Template Set | Pistachio/Bkms_metabolic/Pistachio_ringbreaker/Reaxys/Reaxys_biocatalysis |
Top-N result to add to graph | 6 |
Feasible Synthetic Routes
Q1: What is the molecular formula and weight of PTCDI-C8?
A1: this compound has the molecular formula C44H50N2O4 and a molecular weight of 678.87 g/mol.
Q2: What spectroscopic techniques are used to characterize this compound?
A2: Several techniques are employed, including X-ray photoelectron spectroscopy (XPS) [, , ], ultraviolet photoelectron spectroscopy (UPS) [, ], attenuated total internal reflection Fourier transform infrared (ATR-FTIR) spectroscopy [], and time-resolved photoluminescence (TRPL) []. These techniques provide information about chemical composition, electronic structure, molecular vibrations, and exciton dynamics.
Q3: What is this compound's compatibility with different substrates?
A3: this compound demonstrates compatibility with various substrates, including: * Inorganic Substrates: GaN(0001) [], sapphire [], silicon with native silicon dioxide [], Si/SiO2 [, , , ], and Al2O3 [, , , ].* Organic Substrates: Pentacene [, ], C8-BTBT [], and quaterrylene (QT) [].* Other Substrates: Reduced graphene oxide (rGO) [, ].
Q4: How does temperature affect this compound thin film structure?
A5: this compound thin film structure exhibits temperature dependence. Studies using X-ray diffraction show changes in unit cell parameters with temperature variations. [] A distinct crystal structure is observed at temperatures around 200°C. [, ]
Q5: How does this compound interact with silicon surfaces?
A6: this compound interacts with silicon surfaces through bond formation between its carboxylic groups and silicon dangling bonds, as revealed by XPS. [] This interaction influences the electronic structure at the interface, affecting the HOMO level and work function. [, ]
Q6: What are the primary applications of this compound in organic electronics?
A6: this compound is primarily used as an n-type semiconductor in various organic electronic devices, including:
- Organic Field-Effect Transistors (OFETs): Demonstrates high electron mobility, exceeding that of other organic semiconductors like NTCDA. [, , , , , , , , , ]
- Organic Solar Cells (OSCs): Employed in heterojunction and inverted solar cells, often paired with donor materials like CuPc or P3HT. [, , , , , , ]
- Ring Oscillators: Used in conjunction with p-type organic semiconductors to fabricate ring oscillators, essential components for applications like RFIDs. []
- Sensors: this compound based OFETs show potential as sensors for detecting various analytes, including ions (e.g., Hg2+), biomolecules (e.g., insulin), and environmental parameters (e.g., pH). [, ]
Q7: How does this compound contribute to improving the performance of organic electronic devices?
A8: this compound contributes to device performance through:* High Electron Mobility: Facilitates efficient electron transport, crucial for high current density and switching speeds in OFETs and OSCs. [, , , , ]* Favorable Energy Level Alignment: Enables efficient charge injection and extraction at interfaces with appropriate materials, leading to improved device efficiency. [, , , ]* Crystallinity and Morphology: Formation of well-ordered, crystalline structures enhances charge transport and reduces recombination losses, contributing to improved device performance. [, , , ]
Q8: How does the morphology of this compound influence its function in organic solar cells?
A9: In OSCs, the morphology of this compound is crucial for efficient exciton dissociation and charge transport. [, ] Studies have shown that incorporating this compound nanoribbons into donor polymers enhances device performance by increasing the donor/acceptor interface area for efficient exciton dissociation. []
Q9: What are the current limitations of this compound and areas for future research?
A9: Despite its advantages, further research is needed to address limitations and explore its full potential. Key areas include:
- Improving Air Stability: Investigating new encapsulation techniques or chemical modifications to mitigate the degradation caused by oxygen exposure. [, ]
- Enhancing Charge Carrier Mobility: Exploring strategies like optimized film morphology control, doping, or chemical modifications to further enhance charge transport properties. [, ]
Q10: What are the implications of this compound's interaction with biological systems?
A12: The provided research primarily focuses on the material science aspects of this compound. While some studies explore its application in biosensing [, ], detailed information on its biocompatibility, biodegradability, and potential toxicological effects requires further investigation.
Disclaimer and Information on In-Vitro Research Products
Please be aware that all articles and product information presented on BenchChem are intended solely for informational purposes. The products available for purchase on BenchChem are specifically designed for in-vitro studies, which are conducted outside of living organisms. In-vitro studies, derived from the Latin term "in glass," involve experiments performed in controlled laboratory settings using cells or tissues. It is important to note that these products are not categorized as medicines or drugs, and they have not received approval from the FDA for the prevention, treatment, or cure of any medical condition, ailment, or disease. We must emphasize that any form of bodily introduction of these products into humans or animals is strictly prohibited by law. It is essential to adhere to these guidelines to ensure compliance with legal and ethical standards in research and experimentation.