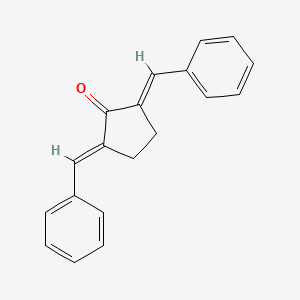
2,5-Dibenzylidenecyclopentanone
Overview
Description
2,5-Dibenzylidenecyclopentanone is a useful research compound. Its molecular formula is C19H16O and its molecular weight is 260.3 g/mol. The purity is usually 95%.
The exact mass of the compound this compound is unknown and the complexity rating of the compound is unknown. The compound has been submitted to the National Cancer Institute (NCI) for testing and evaluation and the Cancer Chemotherapy National Service Center (NSC) number is 629646. The storage condition is unknown. Please store according to label instructions upon receipt of goods.
BenchChem offers high-quality this compound suitable for many research applications. Different packaging options are available to accommodate customers' requirements. Please inquire for more information about this compound including the price, delivery time, and more detailed information at info@benchchem.com.
Scientific Research Applications
Computational Study Insights
A computational study of 2,5-dibenzylidenecyclopentanone revealed insights into its structure and energy isomers. The study found that the lowest energy isomer agrees with spectroscopic and crystallographic data, indicating the compound's stable structure under various conditions (Sanford, Paulisse, & Reeves, 1999).
Spectroscopic Properties
Research on the spectroscopic properties of this compound in acidic media showed evidence for excited-state proton transfer. This suggests its potential in fluorescence studies and as a probe for analyzing environmental changes at the molecular level (Ucak-Astarlioglu & Connors, 2005).
Photodimerisation Studies
Studies on the solid-state photodimerisation of this compound (DBCP) highlighted its unique behavior under UV light, producing distinct dimeric species. This property is valuable for understanding and designing photochemical reactions in solid-state compounds (Theocharis, Jones, Thomas, Motevalli, & Hursthouse, 1984).
Coordination Polymers
This compound has been used to create co-ordination polymers that are photochemically cross-linkable. This suggests applications in materials science where controlled cross-linking and structural modification are necessary (Theocharis, 1987).
Luminescent Characteristics
The luminescent and time-resolved characteristics of this compound and its derivatives have been explored, revealing potential for use in fluorescence studies and photophysical applications. The research showed significant shifts in absorption and fluorescence maxima under different conditions, indicative of its sensitivity to environmental changes (Zakharova et al., 2017).
Crystallography and Molecular Assemblies
Crystallographic studies have been conducted on this compound, providing detailed insights into its molecular structure and assembly. Such studies are crucial for developing materials with specific molecular arrangements and understanding the intermolecular interactions within crystalline structures (Biradha, Nangia, Desiraju, Carrell, & Carrell, 1997).
Conformational Studies
Conformational studies of this compound revealed different isomers within the same crystal lattice. These findings are significant for understanding the polymorphic and conformational behaviors of organic compounds, which are critical in pharmaceuticals and material science (Arshad et al., 2014).
Mechanism of Action
Target of Action
It has been studied for its corrosion inhibition properties on copper in an aqueous sulfuric acid solution .
Mode of Action
The mode of action of 2,5-Dibenzylidenecyclopentanone involves its interaction with copper surfaces in a corrosive environment. The compound acts as a mixed inhibitor due to its adsorption on the copper surface .
Result of Action
It has been shown to inhibit the corrosion of copper in a sulfuric acid solution .
Action Environment
The action, efficacy, and stability of this compound can be influenced by environmental factors. For instance, its corrosion inhibition properties on copper have been studied in an aqueous sulfuric acid solution . The presence of this compound in the solution was found to reduce the rate of copper corrosion .
Biochemical Analysis
Biochemical Properties
2,5-Dibenzylidenecyclopentanone plays a significant role in biochemical reactions due to its ability to interact with various enzymes, proteins, and other biomolecules. It has been shown to inhibit glutathione S-transferases (GSTs), which are enzymes involved in detoxification processes
Cellular Effects
The effects of this compound on cellular processes are profound. It influences cell function by modulating cell signaling pathways, gene expression, and cellular metabolism. Studies have shown that this compound can induce apoptosis in cancer cells by activating the caspase pathway . Additionally, it affects the expression of various genes involved in cell cycle regulation and apoptosis, thereby altering cellular metabolism and promoting cell death in malignant cells.
Molecular Mechanism
At the molecular level, this compound exerts its effects through several mechanisms. It binds to specific biomolecules, leading to enzyme inhibition or activation. For instance, its interaction with GSTs results in the inhibition of these enzymes, thereby affecting the detoxification process . Furthermore, this compound can modulate gene expression by influencing transcription factors and other regulatory proteins, leading to changes in cellular function and metabolism.
Temporal Effects in Laboratory Settings
In laboratory settings, the effects of this compound have been observed to change over time. The compound exhibits stability under various conditions, but its degradation products can also influence cellular function. Long-term studies have shown that prolonged exposure to this compound can lead to sustained inhibition of GSTs and other enzymes, resulting in persistent changes in cellular metabolism and function .
Dosage Effects in Animal Models
The effects of this compound vary with different dosages in animal models. At lower doses, the compound has been shown to inhibit GSTs without causing significant toxicity . At higher doses, it can induce toxic effects, including liver damage and oxidative stress. These findings highlight the importance of determining the optimal dosage for therapeutic applications to minimize adverse effects.
Metabolic Pathways
This compound is involved in various metabolic pathways, interacting with enzymes and cofactors that regulate metabolic flux and metabolite levels. Its inhibition of GSTs affects the detoxification pathway, leading to the accumulation of toxic metabolites . Additionally, the compound can influence other metabolic pathways by modulating the activity of key enzymes involved in cellular metabolism.
Transport and Distribution
The transport and distribution of this compound within cells and tissues are mediated by specific transporters and binding proteins. The compound is known to accumulate in certain tissues, such as the liver, where it exerts its biochemical effects . Its distribution is influenced by factors such as tissue permeability, binding affinity, and the presence of transport proteins.
Subcellular Localization
The subcellular localization of this compound plays a crucial role in its activity and function. The compound is primarily localized in the cytoplasm, where it interacts with various enzymes and proteins . Additionally, it can be targeted to specific subcellular compartments through post-translational modifications and targeting signals, influencing its biochemical activity and cellular effects.
Properties
{ "Design of the Synthesis Pathway": "The synthesis of 2,5-Dibenzylidenecyclopentanone can be achieved through a Claisen-Schmidt condensation reaction between cyclopentanone and benzaldehyde derivatives.", "Starting Materials": [ "Cyclopentanone", "Benzaldehyde", "Sodium hydroxide", "Ethanol" ], "Reaction": [ "Dissolve 10 g of cyclopentanone in 50 mL of ethanol in a round-bottom flask.", "Add 10 g of benzaldehyde to the flask and stir the mixture for 10 minutes.", "Add 5 g of sodium hydroxide to the flask and stir the mixture for 30 minutes.", "Heat the mixture under reflux for 4 hours.", "Cool the mixture to room temperature and filter the precipitate.", "Wash the precipitate with cold ethanol and dry it under vacuum.", "Recrystallize the product from ethanol to obtain 2,5-Dibenzylidenecyclopentanone as a white solid." ] } | |
CAS No. |
895-80-7 |
Molecular Formula |
C19H16O |
Molecular Weight |
260.3 g/mol |
IUPAC Name |
(2Z,5E)-2,5-dibenzylidenecyclopentan-1-one |
InChI |
InChI=1S/C19H16O/c20-19-17(13-15-7-3-1-4-8-15)11-12-18(19)14-16-9-5-2-6-10-16/h1-10,13-14H,11-12H2/b17-13-,18-14+ |
InChI Key |
CVTOCKKPVXJIJK-SIJTWYJSSA-N |
Isomeric SMILES |
C\1C/C(=C/C2=CC=CC=C2)/C(=O)/C1=C/C3=CC=CC=C3 |
SMILES |
C1CC(=CC2=CC=CC=C2)C(=O)C1=CC3=CC=CC=C3 |
Canonical SMILES |
C1CC(=CC2=CC=CC=C2)C(=O)C1=CC3=CC=CC=C3 |
Origin of Product |
United States |
Retrosynthesis Analysis
AI-Powered Synthesis Planning: Our tool employs the Template_relevance Pistachio, Template_relevance Bkms_metabolic, Template_relevance Pistachio_ringbreaker, Template_relevance Reaxys, Template_relevance Reaxys_biocatalysis model, leveraging a vast database of chemical reactions to predict feasible synthetic routes.
One-Step Synthesis Focus: Specifically designed for one-step synthesis, it provides concise and direct routes for your target compounds, streamlining the synthesis process.
Accurate Predictions: Utilizing the extensive PISTACHIO, BKMS_METABOLIC, PISTACHIO_RINGBREAKER, REAXYS, REAXYS_BIOCATALYSIS database, our tool offers high-accuracy predictions, reflecting the latest in chemical research and data.
Strategy Settings
Precursor scoring | Relevance Heuristic |
---|---|
Min. plausibility | 0.01 |
Model | Template_relevance |
Template Set | Pistachio/Bkms_metabolic/Pistachio_ringbreaker/Reaxys/Reaxys_biocatalysis |
Top-N result to add to graph | 6 |
Feasible Synthetic Routes
Q1: What is the molecular formula, weight, and key spectroscopic data for 2,5-dibenzylidenecyclopentanone?
A1: this compound has the molecular formula C19H16O and a molecular weight of 260.33 g/mol. Key spectroscopic data includes characteristic peaks in the IR spectrum for the carbonyl group and C=C bonds. [, ]
Q2: How does the structure of this compound relate to its photochemical reactivity in the solid state?
A2: this compound exhibits photoreactivity in the solid state due to the arrangement of its molecules within the crystal lattice. Even though the reactive double bonds are not perfectly parallel, their close proximity (around 3.7 Å) allows for a [2+2] photodimerization reaction to occur upon UV irradiation. This type of reaction is governed by topochemical principles, where the spatial arrangement of molecules in the solid state dictates the reactivity and stereochemistry of the products. [, , ]
Q3: The solid-state photodimerization of this compound is reported to yield an amorphous product. What is the significance of this observation?
A3: The formation of an amorphous product from a crystalline starting material during the photodimerization of this compound suggests a significant disruption of the original crystal lattice. This implies substantial molecular movement during the reaction, which is unusual for typical topochemical reactions. This unique characteristic could be of interest for applications requiring controlled amorphization or the creation of materials with specific properties. [, ]
Q4: How do substituents on the benzene rings of this compound derivatives affect their photochemical behavior?
A4: The introduction of substituents on the benzene rings of this compound derivatives can dramatically influence their photochemical reactivity in the solid state. For example, the presence of bulky substituents can hinder the [2+2] photodimerization reaction. Additionally, electron-withdrawing or electron-donating groups can affect the electronic properties of the molecule, potentially altering the absorption spectrum and excited-state dynamics, which can influence photoreactivity. [, , ]
Q5: Can you explain the different conformational isomers of this compound observed in its crystal structures?
A5: this compound can exist in different conformational isomers due to the rotation around the single bonds connecting the cyclopentanone ring and the benzylidene groups. These isomers differ in the relative orientation of the phenyl rings and the carbonyl group, leading to variations in their bond lengths, torsion angles, and dihedral angles. Polymorph II of this compound, obtained through slow evaporation from ethanol, uniquely exhibits two conformational isomers within the same crystal lattice. This phenomenon is attributed to the interplay of various intermolecular interactions, including C–H⋯O hydrogen bonds, π-π stacking, and C–H⋯π interactions. []
Q6: How does the alicyclic ring size influence the synthesis of diarylidene and heteroarylidene ketones?
A6: The size of the alicyclic ring significantly impacts the synthesis of diarylidene and heteroarylidene ketones. Smaller rings, like cyclobutanone, are more strained and tend to undergo ring-opening reactions, making the preparation of their corresponding arylidene derivatives more challenging. In contrast, larger rings, like cyclopentanone and cyclohexanone, are less strained and more readily form arylidene derivatives through condensation reactions with aromatic aldehydes. []
Q7: How do this compound and its analogs interact with glutathione S-transferases (GSTs)?
A8: Curcumin, a natural compound structurally related to this compound, exhibits inhibitory activity against human glutathione S-transferases (GSTs), particularly GSTA1-1, GSTM1-1, and GSTP1-1. These enzymes play a crucial role in detoxifying xenobiotics. While many this compound analogs showed weaker inhibitory activity compared to curcumin, certain substitutions, particularly in the B and C series, led to increased potency against specific GST isoenzymes. These findings suggest that modifications to the this compound scaffold can significantly impact GST inhibition and may hold potential for developing new therapeutic agents. []
Q8: What insights do computational studies provide about the electronic structure and properties of this compound?
A9: Computational chemistry techniques, such as DFT calculations, have been employed to investigate the electronic structure and properties of this compound. These studies reveal that the molecule predominantly adopts a trans configuration. Additionally, calculations provide valuable information about the charge distribution, ionization potential, and electron affinity of the molecule, which are crucial parameters for understanding its reactivity and potential applications in materials science. []
Q9: How do modifications to the this compound structure impact its pharmacokinetic properties?
A10: Modifications to the this compound structure can significantly affect its pharmacokinetic properties, including absorption, distribution, metabolism, and excretion (ADME). Computational studies using tools like ADMETsar 2.0 and ADMETlab 2.0 allow researchers to predict the ADME profiles of different analogs. For example, converting the diketone structure of curcumin to the mono-ketone found in this compound analogs can alter drug-likeness properties and interactions with drug transporters and metabolizing enzymes. These modifications can potentially improve bioavailability and other pharmacokinetic parameters, leading to more favorable drug candidates. []
Q10: How is this compound being explored as a potential copper corrosion inhibitor?
A11: Research has explored the potential of this compound and its derivatives as corrosion inhibitors for copper in acidic environments. Electrochemical studies, particularly potentiodynamic polarization measurements, suggest that these compounds can act as mixed-type inhibitors by adsorbing onto the copper surface and hindering the corrosion process. The presence of electron-donating groups, such as dimethylamino substituents, enhances the inhibitory effect, likely due to stronger adsorption to the metal surface. These findings highlight the potential of this compound analogs as environmentally friendly alternatives to traditional copper corrosion inhibitors. [, ]
Q11: What are the potential applications of this compound and its derivatives in coordination polymers?
A12: The ability of this compound to chelate metal ions through its carbonyl and hydroxyl groups makes it a promising building block for coordination polymers. These materials have garnered significant attention due to their potential applications in catalysis, gas storage, and sensing. The photochemical reactivity of some this compound-based coordination polymers makes them particularly intriguing, as it enables post-synthetic modifications that can tailor their properties and functionalities. []
Q12: How do C–H⋯O hydrogen bonds contribute to the supramolecular assembly of this compound?
A13: C–H⋯O hydrogen bonds play a vital role in the formation of supramolecular architectures of this compound and its complexes with molecules like 1,3,5-trinitrobenzene. These non-covalent interactions, though relatively weak, exhibit directionality and can influence the packing arrangement of molecules in the solid state. The carbonyl group of this compound acts as a hydrogen bond acceptor, interacting with the electron-deficient hydrogen atoms of neighboring molecules, leading to the formation of well-defined supramolecular synthons. These interactions contribute to the stability and unique properties of the resulting assemblies. [, ]
Disclaimer and Information on In-Vitro Research Products
Please be aware that all articles and product information presented on BenchChem are intended solely for informational purposes. The products available for purchase on BenchChem are specifically designed for in-vitro studies, which are conducted outside of living organisms. In-vitro studies, derived from the Latin term "in glass," involve experiments performed in controlled laboratory settings using cells or tissues. It is important to note that these products are not categorized as medicines or drugs, and they have not received approval from the FDA for the prevention, treatment, or cure of any medical condition, ailment, or disease. We must emphasize that any form of bodily introduction of these products into humans or animals is strictly prohibited by law. It is essential to adhere to these guidelines to ensure compliance with legal and ethical standards in research and experimentation.