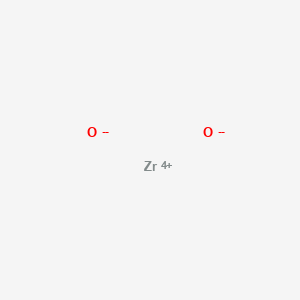
Zirconium oxide
Overview
Description
Zirconium(IV) oxide, also known as zirconia, is a white crystalline oxide of zirconium. It is naturally found in the mineral baddeleyite and is known for its high melting point, thermal stability, and resistance to corrosion. Zirconium(IV) oxide is widely used in various industrial and scientific applications due to its unique properties .
Preparation Methods
Zirconium(IV) oxide can be synthesized through several methods, including:
Sol-Gel Method: This involves the hydrolysis and condensation of zirconium alkoxides, followed by drying and calcination to obtain zirconium(IV) oxide.
Precipitation Method: Zirconium(IV) oxychloride is precipitated using a base such as potassium hydroxide, followed by washing, drying, and calcination.
Hydrothermal Method: This method involves the reaction of zirconium salts with water at high temperatures and pressures to produce zirconium(IV) oxide.
Industrial Production: Industrially, zirconium(IV) oxide is produced by the thermal decomposition of zirconium compounds such as zirconium(IV) chloride or zirconium(IV) sulfate.
Chemical Reactions Analysis
Zirconium(IV) oxide undergoes various chemical reactions, including:
Reduction: When heated with carbon, zirconium(IV) oxide is reduced to zirconium carbide.
Chlorination: When heated with carbon in the presence of chlorine, it converts to zirconium(IV) chloride.
Reaction with Acids: Zirconium(IV) oxide is slowly attacked by concentrated hydrofluoric acid and sulfuric acid.
Common reagents used in these reactions include carbon, chlorine, hydrofluoric acid, and sulfuric acid. The major products formed are zirconium carbide and zirconium(IV) chloride .
Scientific Research Applications
Zirconium(IV) oxide has numerous scientific research applications, including:
Nanocatalysts: Used in various catalytic processes due to its high surface area and stability.
Biomedical Applications: Employed in dentistry for dental implants and crowns due to its biocompatibility and strength.
Drug Delivery: Utilized in drug delivery systems for its ability to carry and release drugs in a controlled manner.
Sensors: Used in gas sensors and biosensors for detecting various analytes.
Thermal Barrier Coatings: Applied in jet and diesel engines to protect components from high temperatures.
Mechanism of Action
The mechanism of action of zirconium(IV) oxide varies depending on its application. In catalysis, zirconium(IV) oxide acts as a support material, providing a high surface area for catalytic reactions. In biomedical applications, its biocompatibility and mechanical properties make it suitable for implants and prosthetics. The molecular targets and pathways involved include interactions with biological tissues and cells, as well as catalytic sites for chemical reactions .
Comparison with Similar Compounds
Zirconium(IV) oxide can be compared with other similar compounds such as:
Titanium(IV) oxide: Both are used in catalysis and biomedical applications, but zirconium(IV) oxide has higher thermal stability.
Hafnium(IV) oxide: Similar in properties to zirconium(IV) oxide, but hafnium(IV) oxide is less commonly used due to its higher cost.
Zirconium(IV) chloride: Used as a catalyst in organic synthesis, but lacks the thermal stability of zirconium(IV) oxide.
Zirconium(IV) oxide is unique due to its combination of high thermal stability, biocompatibility, and resistance to corrosion, making it suitable for a wide range of applications .
Properties
IUPAC Name |
oxygen(2-);zirconium(4+) | |
---|---|---|
Source | PubChem | |
URL | https://pubchem.ncbi.nlm.nih.gov | |
Description | Data deposited in or computed by PubChem | |
InChI |
InChI=1S/2O.Zr/q2*-2;+4 | |
Source | PubChem | |
URL | https://pubchem.ncbi.nlm.nih.gov | |
Description | Data deposited in or computed by PubChem | |
InChI Key |
RVTZCBVAJQQJTK-UHFFFAOYSA-N | |
Source | PubChem | |
URL | https://pubchem.ncbi.nlm.nih.gov | |
Description | Data deposited in or computed by PubChem | |
Canonical SMILES |
[O-2].[O-2].[Zr+4] | |
Source | PubChem | |
URL | https://pubchem.ncbi.nlm.nih.gov | |
Description | Data deposited in or computed by PubChem | |
Molecular Formula |
O2Zr | |
Source | PubChem | |
URL | https://pubchem.ncbi.nlm.nih.gov | |
Description | Data deposited in or computed by PubChem | |
DSSTOX Substance ID |
DTXSID1042520 | |
Record name | Zirconium dioxide | |
Source | EPA DSSTox | |
URL | https://comptox.epa.gov/dashboard/DTXSID1042520 | |
Description | DSSTox provides a high quality public chemistry resource for supporting improved predictive toxicology. | |
Molecular Weight |
123.22 g/mol | |
Source | PubChem | |
URL | https://pubchem.ncbi.nlm.nih.gov | |
Description | Data deposited in or computed by PubChem | |
Physical Description |
Off-white odorless powder; [MSDSonline] | |
Record name | Zirconium oxide | |
Source | Haz-Map, Information on Hazardous Chemicals and Occupational Diseases | |
URL | https://haz-map.com/Agents/8484 | |
Description | Haz-Map® is an occupational health database designed for health and safety professionals and for consumers seeking information about the adverse effects of workplace exposures to chemical and biological agents. | |
Explanation | Copyright (c) 2022 Haz-Map(R). All rights reserved. Unless otherwise indicated, all materials from Haz-Map are copyrighted by Haz-Map(R). No part of these materials, either text or image may be used for any purpose other than for personal use. Therefore, reproduction, modification, storage in a retrieval system or retransmission, in any form or by any means, electronic, mechanical or otherwise, for reasons other than personal use, is strictly prohibited without prior written permission. | |
CAS No. |
53801-45-9, 1314-23-4, 12036-01-0 | |
Record name | Zirconium oxide | |
Source | ChemIDplus | |
URL | https://pubchem.ncbi.nlm.nih.gov/substance/?source=chemidplus&sourceid=0053801459 | |
Description | ChemIDplus is a free, web search system that provides access to the structure and nomenclature authority files used for the identification of chemical substances cited in National Library of Medicine (NLM) databases, including the TOXNET system. | |
Record name | Zirconium dioxide | |
Source | EPA DSSTox | |
URL | https://comptox.epa.gov/dashboard/DTXSID1042520 | |
Description | DSSTox provides a high quality public chemistry resource for supporting improved predictive toxicology. | |
Record name | Zirconium oxide | |
Source | European Chemicals Agency (ECHA) | |
URL | https://echa.europa.eu/substance-information/-/substanceinfo/100.031.656 | |
Description | The European Chemicals Agency (ECHA) is an agency of the European Union which is the driving force among regulatory authorities in implementing the EU's groundbreaking chemicals legislation for the benefit of human health and the environment as well as for innovation and competitiveness. | |
Explanation | Use of the information, documents and data from the ECHA website is subject to the terms and conditions of this Legal Notice, and subject to other binding limitations provided for under applicable law, the information, documents and data made available on the ECHA website may be reproduced, distributed and/or used, totally or in part, for non-commercial purposes provided that ECHA is acknowledged as the source: "Source: European Chemicals Agency, http://echa.europa.eu/". Such acknowledgement must be included in each copy of the material. ECHA permits and encourages organisations and individuals to create links to the ECHA website under the following cumulative conditions: Links can only be made to webpages that provide a link to the Legal Notice page. | |
Record name | Zirconium oxide | |
Source | European Chemicals Agency (ECHA) | |
URL | https://echa.europa.eu/substance-information/-/substanceinfo/100.053.422 | |
Description | The European Chemicals Agency (ECHA) is an agency of the European Union which is the driving force among regulatory authorities in implementing the EU's groundbreaking chemicals legislation for the benefit of human health and the environment as well as for innovation and competitiveness. | |
Explanation | Use of the information, documents and data from the ECHA website is subject to the terms and conditions of this Legal Notice, and subject to other binding limitations provided for under applicable law, the information, documents and data made available on the ECHA website may be reproduced, distributed and/or used, totally or in part, for non-commercial purposes provided that ECHA is acknowledged as the source: "Source: European Chemicals Agency, http://echa.europa.eu/". Such acknowledgement must be included in each copy of the material. ECHA permits and encourages organisations and individuals to create links to the ECHA website under the following cumulative conditions: Links can only be made to webpages that provide a link to the Legal Notice page. | |
Record name | ZIRCONIUM OXIDE | |
Source | FDA Global Substance Registration System (GSRS) | |
URL | https://gsrs.ncats.nih.gov/ginas/app/beta/substances/S38N85C5G0 | |
Description | The FDA Global Substance Registration System (GSRS) enables the efficient and accurate exchange of information on what substances are in regulated products. Instead of relying on names, which vary across regulatory domains, countries, and regions, the GSRS knowledge base makes it possible for substances to be defined by standardized, scientific descriptions. | |
Explanation | Unless otherwise noted, the contents of the FDA website (www.fda.gov), both text and graphics, are not copyrighted. They are in the public domain and may be republished, reprinted and otherwise used freely by anyone without the need to obtain permission from FDA. Credit to the U.S. Food and Drug Administration as the source is appreciated but not required. | |
Synthesis routes and methods I
Procedure details
Synthesis routes and methods II
Procedure details
Synthesis routes and methods III
Procedure details
Disclaimer and Information on In-Vitro Research Products
Please be aware that all articles and product information presented on BenchChem are intended solely for informational purposes. The products available for purchase on BenchChem are specifically designed for in-vitro studies, which are conducted outside of living organisms. In-vitro studies, derived from the Latin term "in glass," involve experiments performed in controlled laboratory settings using cells or tissues. It is important to note that these products are not categorized as medicines or drugs, and they have not received approval from the FDA for the prevention, treatment, or cure of any medical condition, ailment, or disease. We must emphasize that any form of bodily introduction of these products into humans or animals is strictly prohibited by law. It is essential to adhere to these guidelines to ensure compliance with legal and ethical standards in research and experimentation.