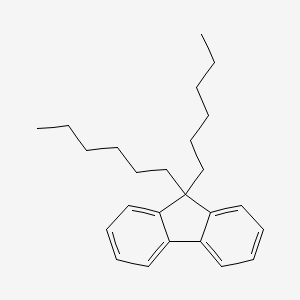
9,9-Dihexylfluorene
Overview
Description
9,9-Dihexylfluorene is a derivative of fluorene, a polycyclic aromatic hydrocarbon. The compound is characterized by the presence of two hexyl groups attached to the ninth carbon of the fluorene structure. This modification enhances its solubility in organic solvents and improves its processability, making it a valuable material in various applications, particularly in the field of organic electronics.
Mechanism of Action
Target of Action
The primary target of 9,9-Dihexylfluorene is the formation of poly (this compound)s (PDHFs) via microwave-assisted Suzuki and Yamamoto coupling reactions . The compound plays a crucial role in the synthesis of these polymers, which have applications in the field of optoelectronics .
Mode of Action
This compound interacts with its targets through a process known as the nonsolvent vapor method (NSVM) . This method allows for the controlled formation of micro- and nanostructures of poly (this compound), including microspheres, nanowires, and butterfly-like structures .
Biochemical Pathways
The biochemical pathways affected by this compound involve the Suzuki and Yamamoto coupling reactions . These reactions are part of the synthesis process of poly (this compound)s, leading to the formation of polymers with higher molecular weights .
Pharmacokinetics
It’s known that the compound’s bioavailability is influenced by the conditions under which it is synthesized, including the use of microwave-assisted polymerization .
Result of Action
The result of this compound’s action is the formation of poly (this compound)s with higher molecular weights . These polymers have potential applications in the field of optoelectronics, including the creation of light-emitting diodes and other devices .
Action Environment
The action of this compound is influenced by environmental factors such as the presence of a nonsolvent vapor . This environment allows for the controlled formation of micro- and nanostructures of poly (this compound), which can be used in the creation of optoelectronic devices .
Biochemical Analysis
Biochemical Properties
These materials have been investigated for their thermal behavior, optical properties, and surface properties
Molecular Mechanism
It’s known that it can participate in Suzuki and Yamamoto coupling reactions to form polyfluorene-based materials . These reactions involve the formation of carbon-carbon bonds and can be facilitated by palladium catalysts .
Metabolic Pathways
It’s known that it can participate in Suzuki and Yamamoto coupling reactions to form polyfluorene-based materials
Preparation Methods
Synthetic Routes and Reaction Conditions: The synthesis of 9,9-Dihexylfluorene typically involves the alkylation of fluorene. One common method is the Friedel-Crafts alkylation, where fluorene reacts with hexyl chloride in the presence of a Lewis acid catalyst such as aluminum chloride. The reaction proceeds as follows:
Fluorene+2Hexyl ChlorideAlCl39,9−Dihexylfluorene+2HCl
Industrial Production Methods: In industrial settings, the production of this compound may involve continuous flow processes to ensure high yield and purity. The use of advanced catalysts and optimized reaction conditions can further enhance the efficiency of the synthesis.
Types of Reactions:
Oxidation: this compound can undergo oxidation reactions, typically forming fluorenone derivatives.
Reduction: Reduction reactions can convert fluorenone derivatives back to this compound.
Substitution: The compound can participate in electrophilic substitution reactions, such as halogenation, nitration, and sulfonation.
Common Reagents and Conditions:
Oxidation: Reagents like potassium permanganate or chromium trioxide in acidic conditions.
Reduction: Reagents like lithium aluminum hydride or sodium borohydride.
Substitution: Halogenation using bromine or chlorine, nitration using nitric acid, and sulfonation using sulfuric acid.
Major Products Formed:
Oxidation: Formation of 9,9-dihexylfluorenone.
Reduction: Regeneration of this compound from its oxidized form.
Substitution: Formation of halogenated, nitrated, or sulfonated derivatives of this compound.
Scientific Research Applications
9,9-Dihexylfluorene has found extensive applications in scientific research due to its unique properties:
Comparison with Similar Compounds
9,9-Dioctylfluorene: Similar to 9,9-Dihexylfluorene but with longer octyl chains, offering different solubility and processing characteristics.
9,9-Diethylfluorene: With shorter ethyl chains, this compound has different solubility and electronic properties compared to this compound.
Uniqueness: this compound stands out due to its balanced solubility and processability, making it a versatile material for various applications. Its hexyl chains provide an optimal balance between flexibility and rigidity, enhancing its performance in organic electronic devices.
Properties
IUPAC Name |
9,9-dihexylfluorene | |
---|---|---|
Source | PubChem | |
URL | https://pubchem.ncbi.nlm.nih.gov | |
Description | Data deposited in or computed by PubChem | |
InChI |
InChI=1S/C25H34/c1-3-5-7-13-19-25(20-14-8-6-4-2)23-17-11-9-15-21(23)22-16-10-12-18-24(22)25/h9-12,15-18H,3-8,13-14,19-20H2,1-2H3 | |
Source | PubChem | |
URL | https://pubchem.ncbi.nlm.nih.gov | |
Description | Data deposited in or computed by PubChem | |
InChI Key |
LQQKFGSPUYTIRB-UHFFFAOYSA-N | |
Source | PubChem | |
URL | https://pubchem.ncbi.nlm.nih.gov | |
Description | Data deposited in or computed by PubChem | |
Canonical SMILES |
CCCCCCC1(C2=CC=CC=C2C3=CC=CC=C31)CCCCCC | |
Source | PubChem | |
URL | https://pubchem.ncbi.nlm.nih.gov | |
Description | Data deposited in or computed by PubChem | |
Molecular Formula |
C25H34 | |
Source | PubChem | |
URL | https://pubchem.ncbi.nlm.nih.gov | |
Description | Data deposited in or computed by PubChem | |
Related CAS |
123863-98-9 | |
Record name | 9H-Fluorene, 9,9-dihexyl-, homopolymer | |
Source | CAS Common Chemistry | |
URL | https://commonchemistry.cas.org/detail?cas_rn=123863-98-9 | |
Description | CAS Common Chemistry is an open community resource for accessing chemical information. Nearly 500,000 chemical substances from CAS REGISTRY cover areas of community interest, including common and frequently regulated chemicals, and those relevant to high school and undergraduate chemistry classes. This chemical information, curated by our expert scientists, is provided in alignment with our mission as a division of the American Chemical Society. | |
Explanation | The data from CAS Common Chemistry is provided under a CC-BY-NC 4.0 license, unless otherwise stated. | |
DSSTOX Substance ID |
DTXSID50408592 | |
Record name | 9,9-dihexylfluorene | |
Source | EPA DSSTox | |
URL | https://comptox.epa.gov/dashboard/DTXSID50408592 | |
Description | DSSTox provides a high quality public chemistry resource for supporting improved predictive toxicology. | |
Molecular Weight |
334.5 g/mol | |
Source | PubChem | |
URL | https://pubchem.ncbi.nlm.nih.gov | |
Description | Data deposited in or computed by PubChem | |
CAS No. |
123863-97-8 | |
Record name | 9,9-dihexylfluorene | |
Source | EPA DSSTox | |
URL | https://comptox.epa.gov/dashboard/DTXSID50408592 | |
Description | DSSTox provides a high quality public chemistry resource for supporting improved predictive toxicology. | |
Synthesis routes and methods I
Procedure details
Synthesis routes and methods II
Procedure details
Retrosynthesis Analysis
AI-Powered Synthesis Planning: Our tool employs the Template_relevance Pistachio, Template_relevance Bkms_metabolic, Template_relevance Pistachio_ringbreaker, Template_relevance Reaxys, Template_relevance Reaxys_biocatalysis model, leveraging a vast database of chemical reactions to predict feasible synthetic routes.
One-Step Synthesis Focus: Specifically designed for one-step synthesis, it provides concise and direct routes for your target compounds, streamlining the synthesis process.
Accurate Predictions: Utilizing the extensive PISTACHIO, BKMS_METABOLIC, PISTACHIO_RINGBREAKER, REAXYS, REAXYS_BIOCATALYSIS database, our tool offers high-accuracy predictions, reflecting the latest in chemical research and data.
Strategy Settings
Precursor scoring | Relevance Heuristic |
---|---|
Min. plausibility | 0.01 |
Model | Template_relevance |
Template Set | Pistachio/Bkms_metabolic/Pistachio_ringbreaker/Reaxys/Reaxys_biocatalysis |
Top-N result to add to graph | 6 |
Feasible Synthetic Routes
Q1: What gives 9,9-Dihexylfluorene its desirable optical properties?
A1: this compound possesses a rigid, planar structure due to its biphenyl core, which allows for efficient π-electron delocalization. This delocalization results in strong absorption and emission in the blue region of the electromagnetic spectrum. [, , , , ]
Q2: How does the presence of this compound affect the energy levels in polymers for organic light-emitting diodes (OLEDs)?
A2: Incorporating this compound into copolymers can raise the highest occupied molecular orbital (HOMO) energy level compared to the corresponding homopolymers. This is particularly useful in OLEDs as it facilitates hole injection from the anode into the polymer layer. []
Q3: Can the emission color of this compound-based polymers be tuned by factors other than copolymerization?
A3: Yes, the emission color of poly[2,7-(this compound)]-block-poly[2-(dimethylamino)ethyl methacrylate] (PF-b-PDMAEMA) can be influenced by factors like solvent composition, temperature, and pH. For instance, varying the water content in a THF solution of PF7-b-PDMAEMA45 leads to a shift in the assembled structure from spheres to cylinders and bundles, ultimately impacting its optical properties. []
Q4: How does the aggregation of this compound units within a polymer matrix impact its fluorescence?
A4: The aggregation of this compound units can lead to fluorescence quenching and a blue shift in the absorption spectra. This phenomenon is observed, for instance, in PF-b-PDMAEMA as the water content is increased, indicating intermolecular interactions between the this compound segments. []
Q5: How do alkyl side chains on the phenylene units of poly(this compound-alt-2,5-dialkoxybenzene) copolymers affect their properties?
A5: Increasing the length of the alkoxy side chains on the phenylene units in poly(this compound-alt-2,5-dialkoxybenzene) copolymers influences their structural organization and properties without significantly altering the electronic structure of the polymer backbone. For example, longer side chains progressively inhibit a melting-recrystallization phenomenon observed during thermal analysis. []
Q6: How does the introduction of carbazole units affect the optical properties of this compound-based copolymers?
A6: Introducing carbazole units into this compound-based copolymers leads to blue-shifted absorption and photoluminescence peaks compared to the poly(this compound) homopolymer. This shift is attributed to the reduced conjugation length caused by the carbazole units. [, ]
Q7: What is the impact of introducing 9-hexylcarbazole and 9-dimethylamionpropylcarbazole moieties into this compound-based copolymers?
A7: The incorporation of these carbazole derivatives effectively suppresses the excimer formation typically observed in poly(this compound). [] This modification can improve the color purity and efficiency of light emission in OLED applications.
Q8: How do bipyridinophane units incorporated into this compound-based copolymers affect their optical and sensory properties?
A8: Bipyridinophane units, when incorporated into this compound copolymers, induce significant red shifts in both absorption and emission spectra due to intramolecular aromatic CH/π interactions. These interactions also enhance the sensitivity of the copolymers to various metal ions, making them potentially useful for sensory applications. []
Q9: What advantages do hyperbranched structures offer in this compound-based polymers compared to their linear counterparts?
A9: Hyperbranched this compound-based polymers exhibit superior properties compared to their linear counterparts. For instance, they demonstrate higher fluorescence efficiency due to the energy funnel effect and enhanced thermal stability with higher decomposition temperatures. [, , ]
Q10: How can the morphology of this compound-based polymers be controlled during processing?
A10: The morphology of this compound-based polymers can be controlled using various techniques. One approach is the nonsolvent vapor method, which has been successfully employed to fabricate microspheres, nanowires, and butterfly-like structures of poly(this compound) by adjusting the solvent and processing conditions. []
Q11: What role does poly(methyl methacrylate) (PMMA) play in the fabrication of this compound-based electrospun nanofibers?
A11: PMMA acts as a supporting matrix in the fabrication of this compound-based electrospun nanofibers. Blending PMMA with poly(this compound) derivatives allows for the creation of nanofibers with controlled diameters and morphologies. The resulting fibers exhibit interesting optical properties due to the aggregation of this compound segments within the PMMA matrix. [, ]
Q12: Can this compound be used in heterojunction structures for photovoltaic applications?
A12: Yes, this compound-based polymers, such as poly(this compound-alt-bithiophene) (F6T2), show promise in organic photovoltaic devices. Blending F6T2 with electron-accepting materials like [, ]-phenyl C61 butyric acid methyl ester (PCBM) can create efficient bulk heterojunction solar cells with promising power conversion efficiencies. [, ]
Q13: How does the morphology of F6T2:PCBM blends impact the performance of organic solar cells?
A13: The morphology of F6T2:PCBM blends plays a crucial role in the performance of organic solar cells. Phase separation within the blend, ideally in the range of 10-20 nm, is crucial for efficient charge transport and exciton dissociation. []
Q14: What are the advantages of using this compound-based polymers in white OLEDs?
A14: this compound-based polymers can be designed to emit white light by incorporating appropriate co-monomers or dopants. Their tunable emission, good charge transport properties, and compatibility with solution processing make them promising candidates for white OLED applications. [, ]
Disclaimer and Information on In-Vitro Research Products
Please be aware that all articles and product information presented on BenchChem are intended solely for informational purposes. The products available for purchase on BenchChem are specifically designed for in-vitro studies, which are conducted outside of living organisms. In-vitro studies, derived from the Latin term "in glass," involve experiments performed in controlled laboratory settings using cells or tissues. It is important to note that these products are not categorized as medicines or drugs, and they have not received approval from the FDA for the prevention, treatment, or cure of any medical condition, ailment, or disease. We must emphasize that any form of bodily introduction of these products into humans or animals is strictly prohibited by law. It is essential to adhere to these guidelines to ensure compliance with legal and ethical standards in research and experimentation.