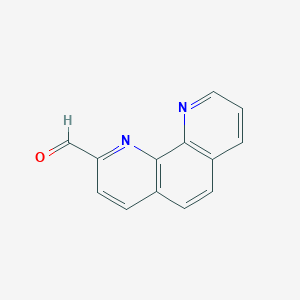
1,10-Phenanthroline-2-carbaldehyde
Overview
Description
1,10-Phenanthroline-2-carbaldehyde is a heterocyclic organic compound with the molecular formula C13H8N2O. It is derived from 1,10-phenanthroline, a well-known ligand in coordination chemistry. The compound is characterized by the presence of an aldehyde group at the second position of the phenanthroline ring system, which imparts unique chemical properties and reactivity.
Mechanism of Action
Target of Action
It is known that phenanthroline derivatives, which include 1,10-phenanthroline-2-carbaldehyde, can form strong complexes with most metal ions . These complexes can interact with various biological targets, influencing their function.
Mode of Action
This compound interacts with its targets primarily through the formation of coordinate bonds and hydrogen bonds . This interaction can lead to changes in the target’s function, potentially influencing various biological processes.
Biochemical Pathways
It has been shown to have anticancer activity , suggesting that it may influence pathways related to cell growth and proliferation.
Result of Action
This compound has been shown to have anticancer activity . It may inhibit the growth of certain types of cancer cells, such as prostate carcinoma cells and human cervical carcinoma cells . This inhibition could be due to the compound’s ability to bind to the DNA of these cells, thereby inhibiting the synthesis of RNA and protein .
Action Environment
The action, efficacy, and stability of this compound can be influenced by various environmental factors. For instance, the pH of the environment could affect the compound’s ability to form coordinate bonds and hydrogen bonds . Additionally, the presence of metal ions in the environment could influence the formation of complexes between this compound and these ions .
Biochemical Analysis
Biochemical Properties
1,10-Phenanthroline-2-carbaldehyde plays a crucial role in biochemical reactions due to its ability to interact with a variety of enzymes, proteins, and other biomolecules. One of its notable interactions is with metal ions, where it acts as a ligand forming strong complexes . This property is particularly useful in studying metalloenzymes, as this compound can inhibit the activity of these enzymes by chelating the metal ions required for their function . Additionally, it has been shown to interact with DNA, stabilizing telomeric quadruplex structures and exhibiting potential antitumor properties .
Cellular Effects
This compound has been observed to exert various effects on different types of cells and cellular processes. In cancer cells, it induces apoptosis by stabilizing telomeric DNA structures, leading to cell cycle arrest and programmed cell death . This compound also influences cell signaling pathways, particularly those involving metal ions, by chelating essential metal cofactors and thereby modulating enzyme activities . Furthermore, this compound affects gene expression by interacting with transcription factors and other DNA-binding proteins .
Molecular Mechanism
The molecular mechanism of action of this compound involves several key interactions at the molecular level. It binds to metal ions, forming stable complexes that inhibit the activity of metalloenzymes . This inhibition occurs through the chelation of metal ions, which are essential cofactors for the catalytic activity of these enzymes . Additionally, this compound can intercalate into DNA, stabilizing specific DNA structures and affecting gene expression . These interactions result in the modulation of various cellular processes, including cell cycle progression and apoptosis .
Temporal Effects in Laboratory Settings
In laboratory settings, the effects of this compound have been studied over different time periods to understand its stability, degradation, and long-term impact on cellular function. The compound is relatively stable under standard laboratory conditions, but its activity can be influenced by factors such as pH and temperature . Over time, this compound may undergo degradation, leading to a decrease in its efficacy . Long-term studies have shown that prolonged exposure to this compound can result in sustained inhibition of metalloenzymes and persistent effects on cellular processes .
Dosage Effects in Animal Models
The effects of this compound vary with different dosages in animal models. At lower doses, the compound exhibits minimal toxicity and effectively inhibits metalloenzyme activity . At higher doses, it can induce toxic effects, including oxidative stress and damage to cellular structures . Threshold effects have been observed, where a specific dosage range is required to achieve the desired biochemical effects without causing significant toxicity . These findings highlight the importance of optimizing dosage levels for therapeutic applications.
Metabolic Pathways
This compound is involved in various metabolic pathways, primarily through its interactions with enzymes and cofactors. It can inhibit the activity of metalloenzymes by chelating metal ions, thereby affecting metabolic flux and altering metabolite levels . Additionally, the compound can influence the expression of genes involved in metabolic processes, further modulating cellular metabolism . These interactions underscore the compound’s potential as a tool for studying metabolic pathways and their regulation.
Transport and Distribution
Within cells and tissues, this compound is transported and distributed through interactions with transporters and binding proteins. The compound’s ability to form stable complexes with metal ions facilitates its transport across cellular membranes . Once inside the cell, it can accumulate in specific compartments, such as the nucleus, where it interacts with DNA and other nuclear proteins . These interactions influence the compound’s localization and its subsequent biochemical effects.
Subcellular Localization
The subcellular localization of this compound is primarily determined by its interactions with specific biomolecules and cellular structures. The compound can be directed to the nucleus, where it binds to DNA and modulates gene expression . Additionally, it may localize to other organelles, such as mitochondria, where it can affect mitochondrial function and cellular metabolism . Post-translational modifications and targeting signals play a role in directing the compound to these specific compartments, influencing its activity and function.
Preparation Methods
Synthetic Routes and Reaction Conditions: 1,10-Phenanthroline-2-carbaldehyde can be synthesized through various methods. One common approach involves the formylation of 1,10-phenanthroline using Vilsmeier-Haack reaction conditions. This reaction typically employs a formylating agent such as N,N-dimethylformamide (DMF) and phosphorus oxychloride (POCl3) to introduce the aldehyde group at the desired position .
Industrial Production Methods: While specific industrial production methods for this compound are not extensively documented, the compound can be produced on a larger scale using optimized versions of the laboratory synthesis methods. The scalability of the Vilsmeier-Haack reaction and other formylation techniques allows for the efficient production of this compound for various applications.
Chemical Reactions Analysis
Types of Reactions: 1,10-Phenanthroline-2-carbaldehyde undergoes several types of chemical reactions, including:
Oxidation: The aldehyde group can be oxidized to a carboxylic acid using oxidizing agents such as potassium permanganate (KMnO4) or chromium trioxide (CrO3).
Reduction: The aldehyde group can be reduced to a primary alcohol using reducing agents like sodium borohydride (NaBH4) or lithium aluminum hydride (LiAlH4).
Substitution: The compound can participate in nucleophilic substitution reactions, where the aldehyde group can be replaced by other functional groups under appropriate conditions.
Common Reagents and Conditions:
Oxidation: Potassium permanganate (KMnO4) in an acidic medium.
Reduction: Sodium borohydride (NaBH4) in methanol or ethanol.
Substitution: Various nucleophiles such as amines or thiols under basic or acidic conditions.
Major Products Formed:
Oxidation: 1,10-Phenanthroline-2-carboxylic acid.
Reduction: 1,10-Phenanthroline-2-methanol.
Substitution: Derivatives of 1,10-phenanthroline with different functional groups at the second position.
Scientific Research Applications
1,10-Phenanthroline-2-carbaldehyde has a wide range of applications in scientific research, including:
Comparison with Similar Compounds
1,10-Phenanthroline: The parent compound, which lacks the aldehyde group at the second position.
2,2’-Bipyridine: Another bidentate ligand similar to 1,10-phenanthroline, but with nitrogen atoms at different positions.
Phenanthrene: The hydrocarbon analog of 1,10-phenanthroline, lacking nitrogen atoms in the ring system.
Uniqueness: 1,10-Phenanthroline-2-carbaldehyde is unique due to the presence of the aldehyde group, which imparts additional reactivity and allows for further functionalization. This makes it a versatile compound for the synthesis of various derivatives and coordination complexes. Its ability to chelate metal ions and participate in diverse chemical reactions distinguishes it from other similar compounds .
Properties
IUPAC Name |
1,10-phenanthroline-2-carbaldehyde | |
---|---|---|
Source | PubChem | |
URL | https://pubchem.ncbi.nlm.nih.gov | |
Description | Data deposited in or computed by PubChem | |
InChI |
InChI=1S/C13H8N2O/c16-8-11-6-5-10-4-3-9-2-1-7-14-12(9)13(10)15-11/h1-8H | |
Source | PubChem | |
URL | https://pubchem.ncbi.nlm.nih.gov | |
Description | Data deposited in or computed by PubChem | |
InChI Key |
SURLLZFZRLZTBI-UHFFFAOYSA-N | |
Source | PubChem | |
URL | https://pubchem.ncbi.nlm.nih.gov | |
Description | Data deposited in or computed by PubChem | |
Canonical SMILES |
C1=CC2=C(C3=C(C=C2)C=CC(=N3)C=O)N=C1 | |
Source | PubChem | |
URL | https://pubchem.ncbi.nlm.nih.gov | |
Description | Data deposited in or computed by PubChem | |
Molecular Formula |
C13H8N2O | |
Source | PubChem | |
URL | https://pubchem.ncbi.nlm.nih.gov | |
Description | Data deposited in or computed by PubChem | |
DSSTOX Substance ID |
DTXSID50406119 | |
Record name | 1,10-phenanthroline-2-carbaldehyde | |
Source | EPA DSSTox | |
URL | https://comptox.epa.gov/dashboard/DTXSID50406119 | |
Description | DSSTox provides a high quality public chemistry resource for supporting improved predictive toxicology. | |
Molecular Weight |
208.21 g/mol | |
Source | PubChem | |
URL | https://pubchem.ncbi.nlm.nih.gov | |
Description | Data deposited in or computed by PubChem | |
Solubility |
>31.2 [ug/mL] (The mean of the results at pH 7.4) | |
Record name | SID49648524 | |
Source | Burnham Center for Chemical Genomics | |
URL | https://pubchem.ncbi.nlm.nih.gov/bioassay/1996#section=Data-Table | |
Description | Aqueous solubility in buffer at pH 7.4 | |
CAS No. |
33795-37-8 | |
Record name | 1,10-phenanthroline-2-carbaldehyde | |
Source | EPA DSSTox | |
URL | https://comptox.epa.gov/dashboard/DTXSID50406119 | |
Description | DSSTox provides a high quality public chemistry resource for supporting improved predictive toxicology. | |
Retrosynthesis Analysis
AI-Powered Synthesis Planning: Our tool employs the Template_relevance Pistachio, Template_relevance Bkms_metabolic, Template_relevance Pistachio_ringbreaker, Template_relevance Reaxys, Template_relevance Reaxys_biocatalysis model, leveraging a vast database of chemical reactions to predict feasible synthetic routes.
One-Step Synthesis Focus: Specifically designed for one-step synthesis, it provides concise and direct routes for your target compounds, streamlining the synthesis process.
Accurate Predictions: Utilizing the extensive PISTACHIO, BKMS_METABOLIC, PISTACHIO_RINGBREAKER, REAXYS, REAXYS_BIOCATALYSIS database, our tool offers high-accuracy predictions, reflecting the latest in chemical research and data.
Strategy Settings
Precursor scoring | Relevance Heuristic |
---|---|
Min. plausibility | 0.01 |
Model | Template_relevance |
Template Set | Pistachio/Bkms_metabolic/Pistachio_ringbreaker/Reaxys/Reaxys_biocatalysis |
Top-N result to add to graph | 6 |
Feasible Synthetic Routes
Q1: What is unique about the interaction of 1,10-Phenanthroline-2-carbaldehyde with Iron(II)?
A1: this compound acts as a tridentate ligand, forming stable complexes with Iron(II). These complexes, particularly when coordinated with phenylhydrazone derivatives, demonstrate a fascinating phenomenon known as spin crossover (SCO). [, , , , ] This means that the Iron(II) ion can transition between a high-spin state (⁵T₂) and a low-spin state (¹A₁) under the influence of external stimuli like temperature or pressure. [, , ]
Q2: What causes the spin crossover behavior in these Iron(II) complexes?
A2: The spin state of the Iron(II) ion within the complex is influenced by the ligand field strength. This compound, when modified with specific substituents, can fine-tune the ligand field strength around the Iron(II) center. [] This delicate balance allows for transitions between high-spin and low-spin states under appropriate conditions.
Q3: Is there evidence of structural changes accompanying the spin crossover transition?
A3: Yes, research using techniques like Mössbauer spectroscopy, magnetic susceptibility measurements, and X-ray diffraction has shown that the spin crossover transition in bis(this compound phenylhydrazone)iron(II) complexes is often accompanied by a crystallographic phase change. [, , ] This suggests a strong coupling between the electronic configuration of the Iron(II) ion and the overall crystal packing.
Q4: How abrupt is this spin crossover transition in these complexes?
A4: The spin crossover in these Iron(II) complexes can be either gradual or abrupt, depending on the specific substituents on the this compound phenylhydrazone ligand. [, ] Furthermore, the transition often exhibits thermal hysteresis, meaning the transition temperature observed during cooling differs from that during heating. [, ] This hysteresis behavior is indicative of cooperative effects within the crystal lattice.
Q5: How does pressure affect the spin crossover behavior?
A5: Studies have shown that applying pressure to these complexes generally widens the thermal hysteresis loop. [] This effect is attributed to pressure influencing the cooperative interactions within the crystal structure, thereby affecting the energy barrier between the high-spin and low-spin states.
Q6: What is the significance of studying the thermodynamics and kinetics of spin crossover in solution?
A6: Understanding the solution behavior of these complexes provides valuable insights into the factors governing spin crossover dynamics in environments more relevant to biological systems. [] Techniques like variable temperature ¹H NMR spectroscopy have proven useful for determining thermodynamic parameters (like transition temperature) and kinetic parameters (like rate constants) of the spin crossover process. []
Disclaimer and Information on In-Vitro Research Products
Please be aware that all articles and product information presented on BenchChem are intended solely for informational purposes. The products available for purchase on BenchChem are specifically designed for in-vitro studies, which are conducted outside of living organisms. In-vitro studies, derived from the Latin term "in glass," involve experiments performed in controlled laboratory settings using cells or tissues. It is important to note that these products are not categorized as medicines or drugs, and they have not received approval from the FDA for the prevention, treatment, or cure of any medical condition, ailment, or disease. We must emphasize that any form of bodily introduction of these products into humans or animals is strictly prohibited by law. It is essential to adhere to these guidelines to ensure compliance with legal and ethical standards in research and experimentation.