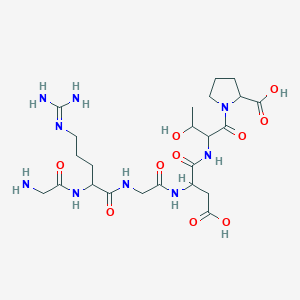
Grgdtp
Overview
Description
This compound plays a crucial role in cell adhesion processes, particularly in the interaction with integrins, which are transmembrane receptors that facilitate cell-extracellular matrix adhesion.
Preparation Methods
Synthetic Routes and Reaction Conditions
The synthesis of Grgdtp typically involves solid-phase peptide synthesis (SPPS), a method widely used for the production of peptides. The process begins with the attachment of the first amino acid to a solid resin. Subsequent amino acids are added sequentially through a series of coupling and deprotection steps. The reaction conditions often involve the use of coupling reagents such as N,N’-diisopropylcarbodiimide (DIC) and hydroxybenzotriazole (HOBt) to facilitate peptide bond formation. The final product is cleaved from the resin and purified using high-performance liquid chromatography (HPLC) .
Industrial Production Methods
In an industrial setting, the production of this compound can be scaled up using automated peptide synthesizers. These machines automate the repetitive steps of SPPS, allowing for the efficient and large-scale production of peptides. The use of advanced purification techniques, such as preparative HPLC, ensures the high purity of the final product .
Chemical Reactions Analysis
Types of Reactions
Grgdtp can undergo various chemical reactions, including:
Oxidation: The oxidation of the thiol group in cysteine residues (if present) can lead to the formation of disulfide bonds.
Reduction: Reduction reactions can break disulfide bonds, converting them back to thiol groups.
Substitution: Amino acid residues in this compound can be substituted with other amino acids to create analogs with different properties.
Common Reagents and Conditions
Oxidation: Hydrogen peroxide (H2O2) or iodine (I2) can be used as oxidizing agents.
Reduction: Dithiothreitol (DTT) or tris(2-carboxyethyl)phosphine (TCEP) are common reducing agents.
Substitution: Amino acid derivatives and coupling reagents like DIC and HOBt are used in substitution reactions.
Major Products Formed
The major products formed from these reactions depend on the specific modifications made to the peptide. For example, oxidation can lead to the formation of disulfide-linked dimers, while substitution can result in analogs with altered biological activity .
Scientific Research Applications
Grgdtp has a wide range of applications in scientific research:
Chemistry: Used as a model peptide for studying peptide synthesis and conformational analysis.
Biology: Plays a role in cell adhesion studies, particularly in the interaction with integrins.
Medicine: Investigated for its potential in drug delivery systems and as a therapeutic agent in wound healing and tissue engineering.
Industry: Utilized in the development of biomaterials and coatings that promote cell adhesion
Mechanism of Action
Grgdtp exerts its effects primarily through its interaction with integrins. Integrins are transmembrane receptors that mediate cell-extracellular matrix adhesion. The arginine-glycine-aspartic acid (RGD) sequence within this compound is recognized by integrins, facilitating the binding of cells to the extracellular matrix. This interaction triggers intracellular signaling pathways that regulate cell migration, proliferation, and survival .
Comparison with Similar Compounds
Similar Compounds
RGD Peptide: Composed of arginine, glycine, and aspartic acid, this peptide is also recognized by integrins and plays a role in cell adhesion.
RGDS Peptide: Similar to RGD but with an additional serine residue, enhancing its binding affinity to certain integrins.
RGD-K Peptide: Contains a lysine residue, which can be used for conjugation to other molecules or surfaces
Uniqueness of Grgdtp
This compound is unique due to its specific sequence, which includes threonine and proline in addition to the RGD motif. This unique sequence enhances its binding specificity and affinity to certain integrins, making it a valuable tool in cell adhesion studies and therapeutic applications .
Properties
IUPAC Name |
1-[2-[[2-[[2-[[2-[(2-aminoacetyl)amino]-5-(diaminomethylideneamino)pentanoyl]amino]acetyl]amino]-3-carboxypropanoyl]amino]-3-hydroxybutanoyl]pyrrolidine-2-carboxylic acid | |
---|---|---|
Source | PubChem | |
URL | https://pubchem.ncbi.nlm.nih.gov | |
Description | Data deposited in or computed by PubChem | |
InChI |
InChI=1S/C23H39N9O10/c1-11(33)18(21(40)32-7-3-5-14(32)22(41)42)31-20(39)13(8-17(36)37)30-16(35)10-28-19(38)12(29-15(34)9-24)4-2-6-27-23(25)26/h11-14,18,33H,2-10,24H2,1H3,(H,28,38)(H,29,34)(H,30,35)(H,31,39)(H,36,37)(H,41,42)(H4,25,26,27) | |
Source | PubChem | |
URL | https://pubchem.ncbi.nlm.nih.gov | |
Description | Data deposited in or computed by PubChem | |
InChI Key |
UMZVBZDHGKJFGQ-UHFFFAOYSA-N | |
Source | PubChem | |
URL | https://pubchem.ncbi.nlm.nih.gov | |
Description | Data deposited in or computed by PubChem | |
Canonical SMILES |
CC(C(C(=O)N1CCCC1C(=O)O)NC(=O)C(CC(=O)O)NC(=O)CNC(=O)C(CCCN=C(N)N)NC(=O)CN)O | |
Source | PubChem | |
URL | https://pubchem.ncbi.nlm.nih.gov | |
Description | Data deposited in or computed by PubChem | |
Molecular Formula |
C23H39N9O10 | |
Source | PubChem | |
URL | https://pubchem.ncbi.nlm.nih.gov | |
Description | Data deposited in or computed by PubChem | |
DSSTOX Substance ID |
DTXSID60405178 | |
Record name | GRGDTP | |
Source | EPA DSSTox | |
URL | https://comptox.epa.gov/dashboard/DTXSID60405178 | |
Description | DSSTox provides a high quality public chemistry resource for supporting improved predictive toxicology. | |
Molecular Weight |
601.6 g/mol | |
Source | PubChem | |
URL | https://pubchem.ncbi.nlm.nih.gov | |
Description | Data deposited in or computed by PubChem | |
CAS No. |
108682-58-2 | |
Record name | GRGDTP | |
Source | EPA DSSTox | |
URL | https://comptox.epa.gov/dashboard/DTXSID60405178 | |
Description | DSSTox provides a high quality public chemistry resource for supporting improved predictive toxicology. | |
Retrosynthesis Analysis
AI-Powered Synthesis Planning: Our tool employs the Template_relevance Pistachio, Template_relevance Bkms_metabolic, Template_relevance Pistachio_ringbreaker, Template_relevance Reaxys, Template_relevance Reaxys_biocatalysis model, leveraging a vast database of chemical reactions to predict feasible synthetic routes.
One-Step Synthesis Focus: Specifically designed for one-step synthesis, it provides concise and direct routes for your target compounds, streamlining the synthesis process.
Accurate Predictions: Utilizing the extensive PISTACHIO, BKMS_METABOLIC, PISTACHIO_RINGBREAKER, REAXYS, REAXYS_BIOCATALYSIS database, our tool offers high-accuracy predictions, reflecting the latest in chemical research and data.
Strategy Settings
Precursor scoring | Relevance Heuristic |
---|---|
Min. plausibility | 0.01 |
Model | Template_relevance |
Template Set | Pistachio/Bkms_metabolic/Pistachio_ringbreaker/Reaxys/Reaxys_biocatalysis |
Top-N result to add to graph | 6 |
Feasible Synthetic Routes
A: GRGDTP competitively binds to integrin receptors, primarily those recognizing the Arginine-Glycine-Aspartic Acid (RGD) motif present in various extracellular matrix (ECM) proteins like fibronectin, vitronectin, and collagen. [, , ]
ANone: By blocking integrin-ECM interactions, this compound can inhibit a wide range of cellular processes, including:
- Cell adhesion: This compound effectively disrupts cell adhesion to ECM proteins, influencing cell spreading and attachment. [, , , ]
- Cell migration: Disruption of integrin function by This compound significantly impairs cell migration, especially in processes requiring interaction with ECM components like tumor cell invasion. [, , , ]
- Signal transduction: This compound binding interferes with integrin-mediated signal transduction pathways, affecting processes like cell proliferation, differentiation, and survival. [, , , , , ]
- Phagocytosis: This compound can modulate collagen phagocytosis by fibroblasts, a crucial process for ECM degradation. This effect suggests the involvement of α2 integrin in this process. []
ANone: this compound has a molecular formula of C26H42N8O11 and a molecular weight of 646.66 g/mol.
A: Yes, ¹H-NMR studies reveal the presence of a weak 5→2 hydrogen-bonded β-turn type-III conformation for This compound in solution. Molecular dynamics simulations suggest the coexistence of inverse γ-turn (3→1) and γ-turn (4→2) conformations. These findings indicate a degree of conformational flexibility within the This compound molecule. []
ANone: The provided research papers don't specifically focus on this compound's material compatibility or stability under various conditions. This aspect might require further investigation, especially when considering its potential use in diverse applications.
ANone: this compound is not reported to have inherent catalytic properties. Its primary mode of action relies on competitive binding to integrins, rather than catalyzing chemical reactions.
A: Yes, molecular dynamics simulations have been used to explore the conformational flexibility of This compound. [] Further computational studies, such as docking simulations or quantitative structure-activity relationship (QSAR) modeling, could provide deeper insights into its interaction with different integrin subtypes and guide the development of more potent and selective analogs.
A: Research demonstrates that even minor alterations in the This compound sequence can significantly impact its biological activity. For example, substituting Aspartic acid with Glutamic acid or altering the peptide's cyclic structure can significantly reduce its inhibitory potency towards integrins. [, ] This sensitivity to structural changes highlights the importance of precise molecular interactions for effective integrin inhibition.
ANone: The research papers provided do not delve into the specific stability profile of this compound or discuss formulation strategies for enhancing its properties. Further research is needed to explore these aspects, particularly if considering this compound for therapeutic applications.
ANone: As this compound is primarily a research tool, specific SHE regulations pertaining to its handling, use, and disposal might vary depending on the institution and local guidelines. It's always crucial to consult relevant safety data sheets and follow appropriate laboratory practices.
ANone: Detailed information on the absorption, distribution, metabolism, and excretion (ADME) of this compound and its in vivo activity and efficacy requires further investigation.
A: Numerous in vitro studies demonstrate the efficacy of This compound in inhibiting cell adhesion, migration, and specific signaling pathways. [, , , , , , , , , , , , , ] Animal models would be valuable for exploring the therapeutic potential and safety profile of This compound in vivo.
Disclaimer and Information on In-Vitro Research Products
Please be aware that all articles and product information presented on BenchChem are intended solely for informational purposes. The products available for purchase on BenchChem are specifically designed for in-vitro studies, which are conducted outside of living organisms. In-vitro studies, derived from the Latin term "in glass," involve experiments performed in controlled laboratory settings using cells or tissues. It is important to note that these products are not categorized as medicines or drugs, and they have not received approval from the FDA for the prevention, treatment, or cure of any medical condition, ailment, or disease. We must emphasize that any form of bodily introduction of these products into humans or animals is strictly prohibited by law. It is essential to adhere to these guidelines to ensure compliance with legal and ethical standards in research and experimentation.